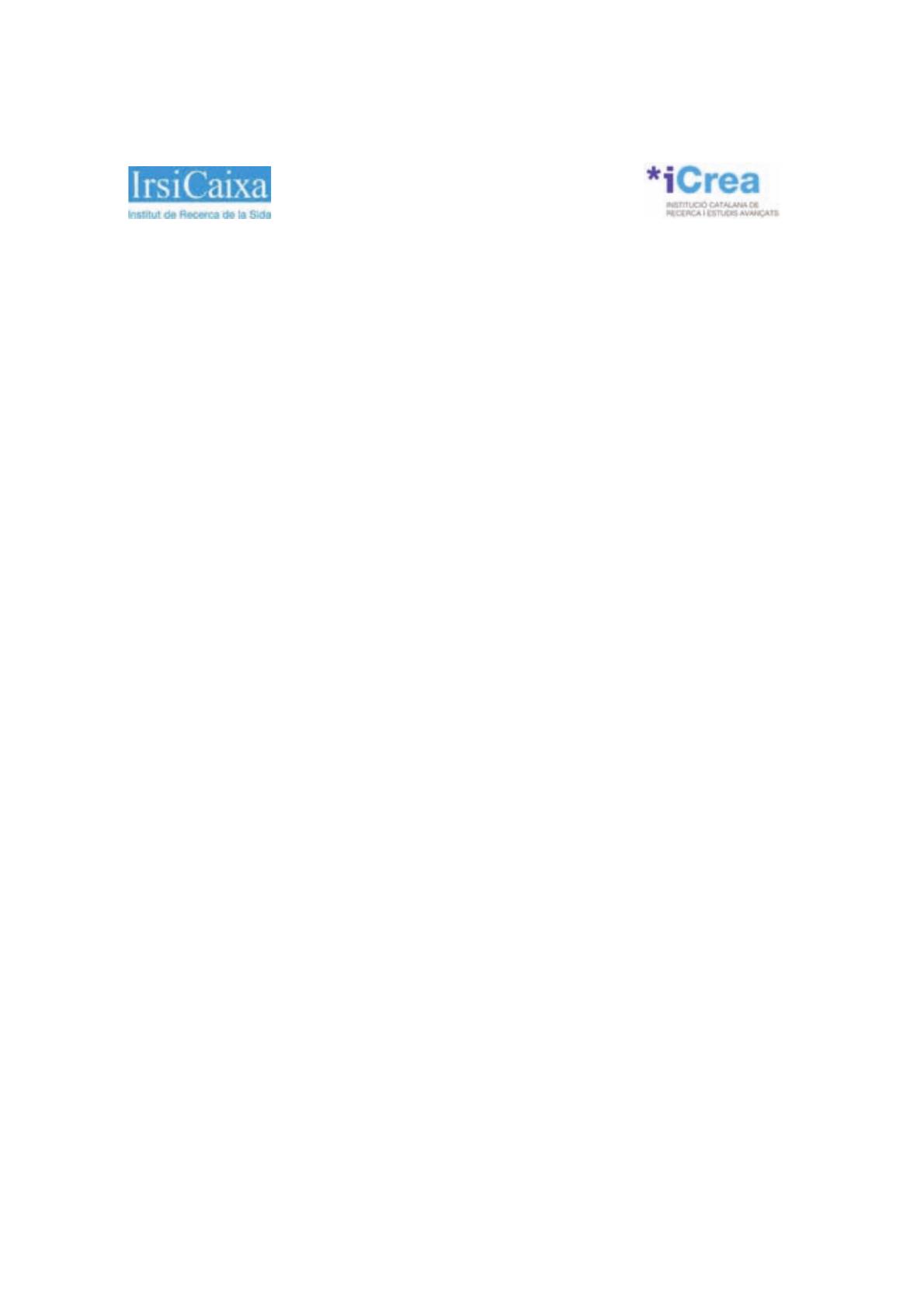
El doctor Javier Martínez Picado, investigador principal i professor de recerca
ICREA a l’Institut de Recerca de la Sida - IrsiCaixa (Hospital U. Germans Trias i
Pujol de Badalona),
Certifica:
Que el treball experimental realitzat i la redacció de la memòria de la Tesi Doctoral
titulada “Viral and host factors involved in rapid HIV-1 disease progression” han
estat realitzats per na Judith Dalmau Moreno sota la seva direcció i considera que
és apte per a ser presentat per a optar al grau de Doctor en Bioquímica, Biologia
Molecular i Biomedicina per la Universitat Autònoma de Barcelona.
I per tal que en quedi constància, signa aquest document a Badalona, 30 de Gener
del 2014.
Prof. Javier Martínez-Picado