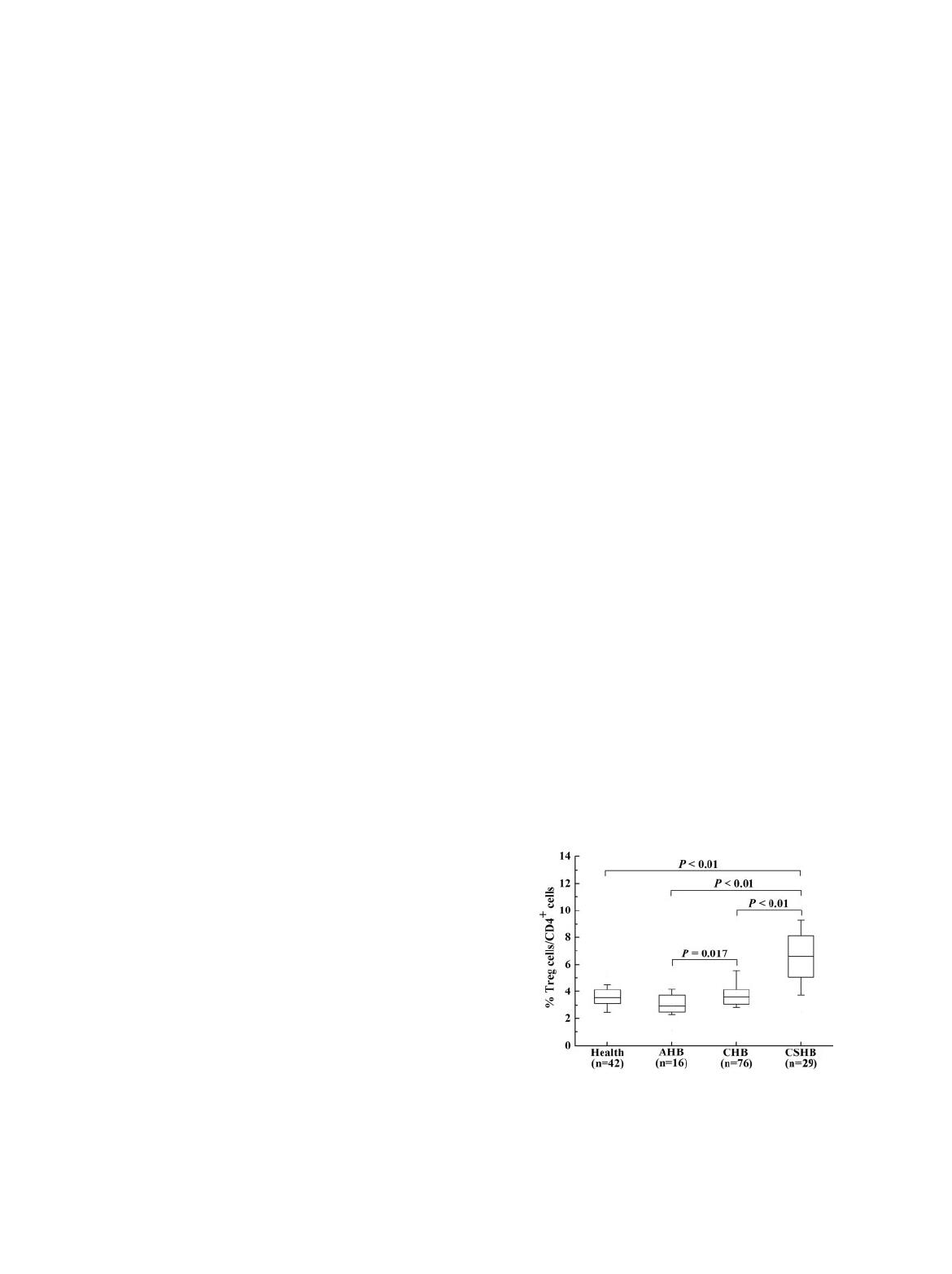
manufacturer’s instructions. For proliferation test, the plate was cultured at
the same condition for 5 days, with replenishment of fresh medium con-
taining 20 U/ml recombinant human (rh) IL-2 (EuroCetus) at day 3. For the
last 18 h the cultures were pulsed with 0.5
Ci/well [
3
H]thymidine and
harvested using a multichannel harvester. The amount of incorporated
[
3
H]thymidine was determined by liquid scintillation spectroscopy. Per-
centage suppression of cellular proliferation by depletion of CD4
⫹
CD25
⫹
Treg from PBMC or by adding of CD4
⫹
CD25
⫹
Treg to PBMC-Treg was
calculated using the formula: 1 ⫺(cpm in the presence of CD4
⫹
CD25
⫹
Treg/(cpm in the absence of CD4
⫹
CD25
⫹
Treg) ⫻100%.
Virological assessment
HBsAg, anti-HBs, total and IgM anti-HBc, hepatitisBeAg(HBeAg),
anti-HBe, anti-HCV, anti-HDV, anti-HGV and anti-HIV were determined
by commercial enzyme immunoassay kits (Kewei Diagnostic), respec-
tively. Serum HBV DNA was quantified by using a commercial real-time
PCR kit (PG Biotech) according to the manufacturer’s instruction. HBV
DNA detection limit threshold is 5 ⫻10
2
copies/ml.
Immunohistochemical staining of paraffin-embedded liver tissue
Paraffin-embedded, formalin-fixed liver tissue was cut into 5-
m sections
and placed on polylysine-coated slides. Slides were processed for immu-
nohistochemistry as previously described (42). Ag retrieval was achieved
via pressure cooking for 3 min in citrate buffer (pH 6.0). mAb of anti-
human CD4 (Vector Laboratories), CD8 (DakoCytomation) and biotinyl-
ated goat anti-mouse Ig (Zhongshan Goldenbridge Biotech) were used for
CD4 and CD8 staining, respectively. Goat-anti-human FoxP3 (Abcam) and
biotinylated rabbit anti-goat Ig were used for FoxP3 staining. The slides
were stained with hematoxylin following immunohistochemical staining.
For the enumeration of positive lymphocytes, lymphocytes were counted in
three high-powered fields (hpf; ⫻400) by two independent observers. Low-
powered fields (lpf; ⫻100) were used for counting FoxP3
⫹
cells because of
less abundance of the cells in the liver. For each sample, the mean per-
centage of positive cells was taken. Results were expressed as the median
and range of all tested patents in each group.
Statistic analysis
All data were analyzed using SPSS version 13.0 for Windows software.
The Kruskal-Wallis H test and Mann-Whitney nonparametric Utest were
used for comparison between groups. Bonferroni step down (Holm) cor-
rection was applied when multiple comparisons were made. Spearman cor-
relation analysis was performed between the frequency of CD4
⫹
CD25
⫹
Treg and other parameters. Value of p⬍0.05 is considered as a significant
difference.
Results
Phenotypic characterization of CD4
⫹
CD25
⫹
Treg
As illustrated in Fig. 1c, the CD4
⫹
CD25
high
subpopulation ex-
pressed a significantly higher level of intracellular FoxP3 and
CTLA-4, surface HLA-DR, CD45RO, and CD95 but a lower level
of surface CD45RA compared with CD4
⫹
CD25
low
or
CD4
⫹
CD25
⫺
subpopulations. CD4
⫹
CD25
low
subpopulation ex-
pressed significantly higher levels of intracellular CTLA-4 and
FoxP3, surface CD45RO, and CD95 but similar levels of HLA-DR
and lower levels of surface CD45RA than CD4
⫹
CD25
⫺
subpopu-
lation. FoxP3 expression was highly enriched in CD4
⫹
CD25
high
subpopulation (96%) rather than subpopulations of CD4
⫹
CD25
low
(27%) and CD4
⫹
CD25
⫺
(2.1%) which is consistent with the cur-
rent description of Treg. There is no significant difference in the
phenotypic profile of CD4
⫹
CD25
⫹
Treg among patients with
AHB, CHB, CSHB, and healthy controls (data not shown).
FoxP3 mRNA is selectively expressed in CD4
⫹
CD25
⫹
Treg
Because FoxP3 was reported as a unique marker primarily re-
stricted to CD4
⫹
CD25
⫹
Treg (16), we measured FoxP3 mRNA
levels in HBV-infected patients with different disease statuses. To
determine the fractional abundance of FoxP3 mRNA in various
cell subsets, RNA extracted from PBMC and isolated CD4
⫺
,
CD4
⫹
, CD4
⫹
CD25
⫺
, and CD4
⫹
CD25
⫹
cell subsets were indi-
vidually subjected to real-time PCR to quantify FoxP3 expression.
The results showed the expression indexes of FoxP3 mRNA were
2.3, 0.4, 11.2, and 64.0 for PBMC, CD4
⫺
, CD4
⫹
, and
CD4
⫹
CD25
⫹
subpopulations, respectively (Fig. 1d). FoxP3
mRNA level in CD4
⫹
CD25
⫹
Treg was 63-fold higher than the
CD4
⫹
CD25
⫺
cell subset, suggesting FoxP3 mRNA expression
was highly enriched in CD4
⫹
CD25
⫹
Treg. Considering the limi-
tation of obtaining blood specimen in clinic, we measured FoxP3
mRNA expression from total CD4
⫹
T cells rather than the isolated
CD4
⫹
CD25
⫹
Treg. The results showed that the mean relative
FoxP3 mRNA levels of CD4
⫹
T cells in healthy controls, AHB
patients, CHB patients, and CSHB patients were 0.056 ⫾0.021,
0.053 ⫾0.017, 0.059 ⫾0.053, and 0.199 ⫾0.174, respectively
(Fig. 1e). Therefore, FoxP3 mRNA is selectively expressed in
CD4
⫹
CD25
⫹
Treg and CSHB patients have higher levels of
FoxP3 mRNA.
Increased circulating CD4
⫹
CD25
⫹
Treg frequencies in chronic
severe HBV-infected patients
Studies with humans have shown that the majority of CD4
⫹
T cells
with regulatory function are segregated to the CD25
high
subset
(40), and the CD4
⫹
CD25
high
subset exhibits more homogeneous
characteristics of Treg than CD4
⫹
CD25
low
subset (14). The
CD4
⫹
CD25
high
subset has been counted to represent the
CD4
⫹
CD25
⫹
Treg frequency in HBV and HIV-infected patients
(35, 43). We thus determined the frequency of CD4
⫹
CD25
⫹
Treg
by measuring CD4
⫹
CD25
high
subset (Fig. 2). Circulating
CD4
⫹
CD25
⫹
Treg frequency in CSHB patients has not been de-
scribed previously. Our study showed that the frequency of the
circulating Treg in these patients was significantly higher than
healthy controls (CSHB patients vs healthy controls, median
6.59%, mean 6.65%, SD, 2.01 vs 3.57%, 3.60 ⫾0.75%, p⬍0.01),
CHB patients (vs 3.74%, 3.90 ⫾1.41%, p⬍0.01), and AHB
patients in the early acute phase (week 1) of their illness (vs
3.02%, 3.10 ⫾0.87%, p⬍0.01). CHB patients had a higher per-
centage of circulating CD4
⫹
CD25
⫹
Treg than early acute phase
AHB patients ( p⫽0.017), but had no significant difference com-
pared with healthy controls. The comparison of circulating
CD4
⫹
CD25
⫹
Treg frequencies between early acute phase AHB
patients and healthy controls exhibited no significant difference
(Fig. 2). We have also noticed that increased numbers of higher
Treg was clinically associated with more severe illness of CSHB.
FIGURE 2. The frequency of CD4
⫹
CD25
⫹
Treg in peripheral blood of
various subjects. Data are expressed as box plots, in which the horizontal
lines illustrate the 25th, 50th, and 75th percentiles of the frequencies of
CD4
⫹
CD25
⫹
Treg as measured by flow cytometry. The vertical lines rep-
resent the 10th and 90th percentiles. The pvalues for multiple comparisons
were calculated by using the Kruskal-Wallis H nonparametric test. AHB
patients were sampled in the early acute phase (week 1) of their illness.
Value of p⬍0.001 for multiple comparisons by Kruskal-Wallis H non-
parametric test.
742 CHARACTERIZATION OF Treg IN HBV INFECTION