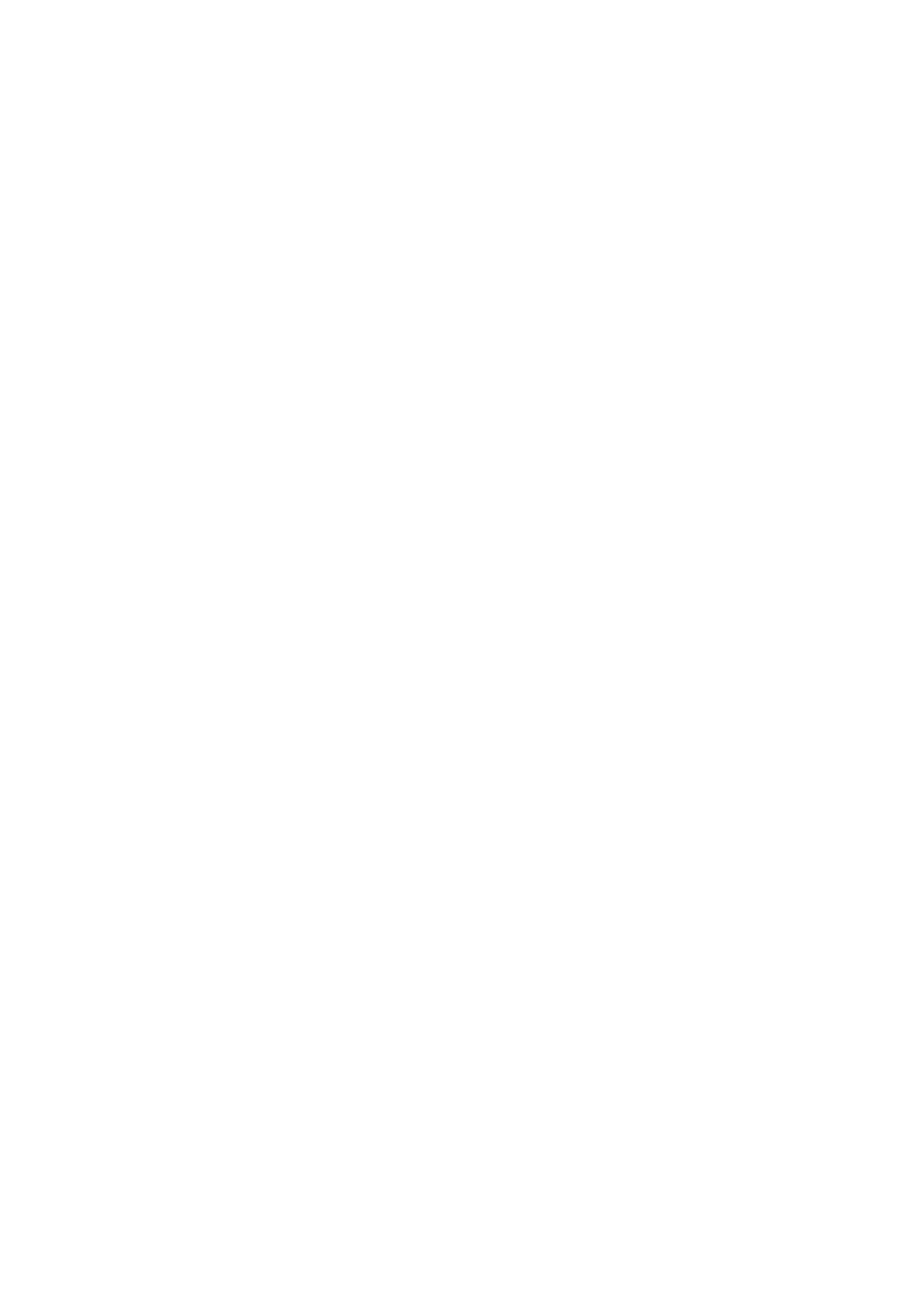
4
Agradecimentos
À Profa. Dra. Ana Luiza Maia, os meus agradecimentos pela orientação, pela
confiança, pela presença segura e estimulante. Agradeço a amizade que se
consolidou nesses quase 7 anos de ótima convivência.
Às amigas e colegas do Laboratório de Biologia Molecular – Grupo de
Tireóide: Márcia Punãles, Lenara Golbert, Simone Wajner, Clarissa Capp, Márcia
Wagner, Andréia Possatti da Rocha, Débora Siqueira e Aline Estivallet, por
proporcionarem tão agradável ambiente de trabalho.
Aos bolsistas e ex-bolsistas de iniciação científica, em especial ao residente
Miguel Dora e ao acadêmico Iuri Goemann pelo auxílio na condução das pesquisas.
Aos Profs. Dr. Alceu Migliavacca e Dr. José Ricardo Guimarães, pela
colaboração na coleta das amostras para o estudo.
Às Profas. Dra. Edna Kimura e Dra. Mary Cleide Sogayar da Universidade de
São Paulo pelo fornecimento da linhagem celular, fundamental para o seguimento
dos experimentos.
Ao pessoal do Centro de Terapia Gênica do Hospital de Clínicas de Porto
Alegre, representados pela Drª. Úrsula Matte, pelas orientações quanto à técnica de
cultivo celular.
Aos preceptores do Serviço de Endocrinologia do Hospital São Lucas da
Pontifícia Universidade Católica do Rio Grande do Sul, onde iniciei minha prática
clínica em Endocrinologia.
Ao meu marido Bernard F Meyer, por todo o seu amor e carinho. Agradeço
por seres um grande pai.