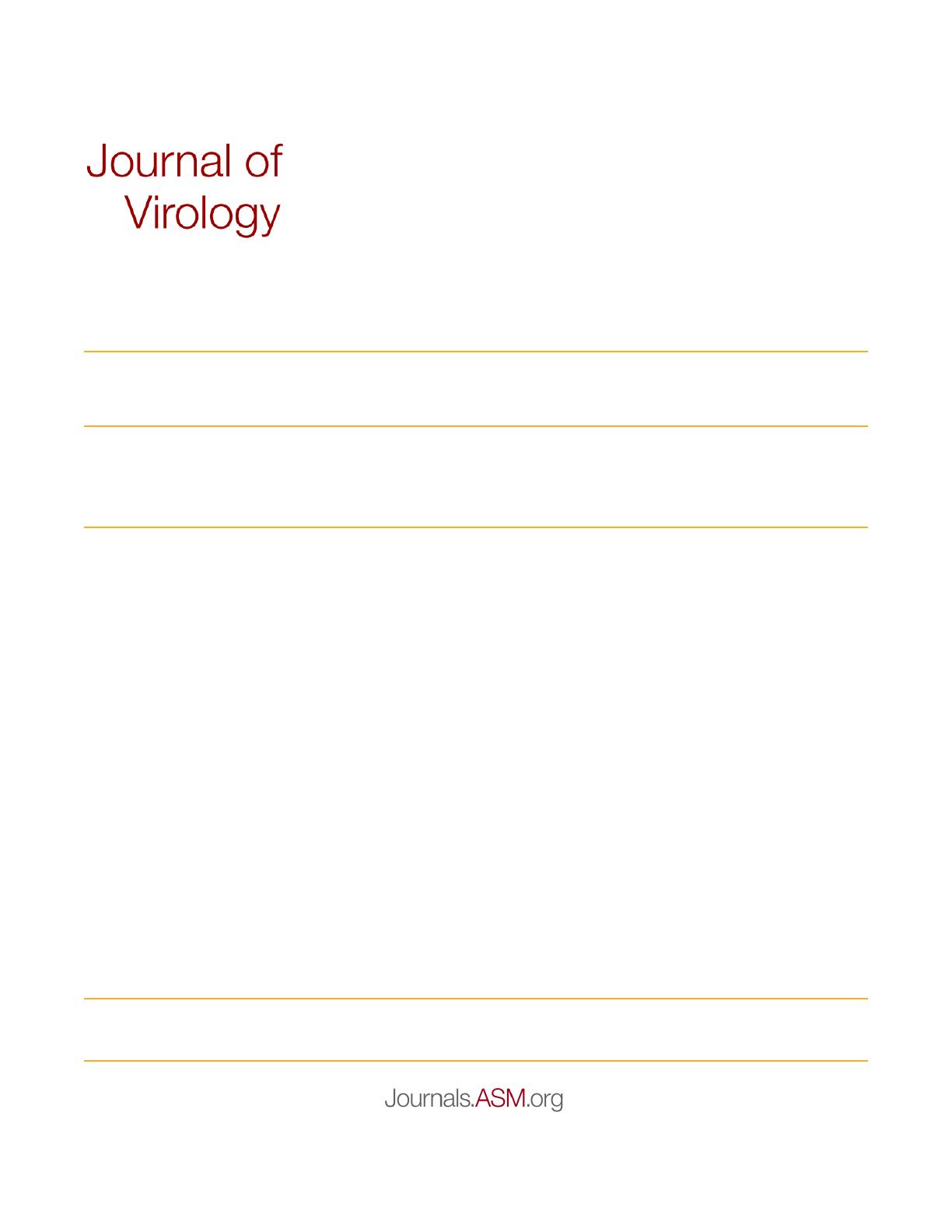
1992, 66(3):1476. J. Virol.
K Tsukiyama-Kohara, N Iizuka, M Kohara and A Nomoto
virus RNA.
Internal ribosome entry site within hepatitis C
http://jvi.asm.org/content/66/3/1476
Updated information and services can be found at:
These include:
CONTENT ALERTS more»cite this article),
Receive: RSS Feeds, eTOCs, free email alerts (when new articles
http://journals.asm.org/site/misc/reprints.xhtmlInformation about commercial reprint orders: http://journals.asm.org/site/subscriptions/To subscribe to to another ASM Journal go to:
on February 23, 2013 by PENN STATE UNIVhttp://jvi.asm.org/Downloaded from