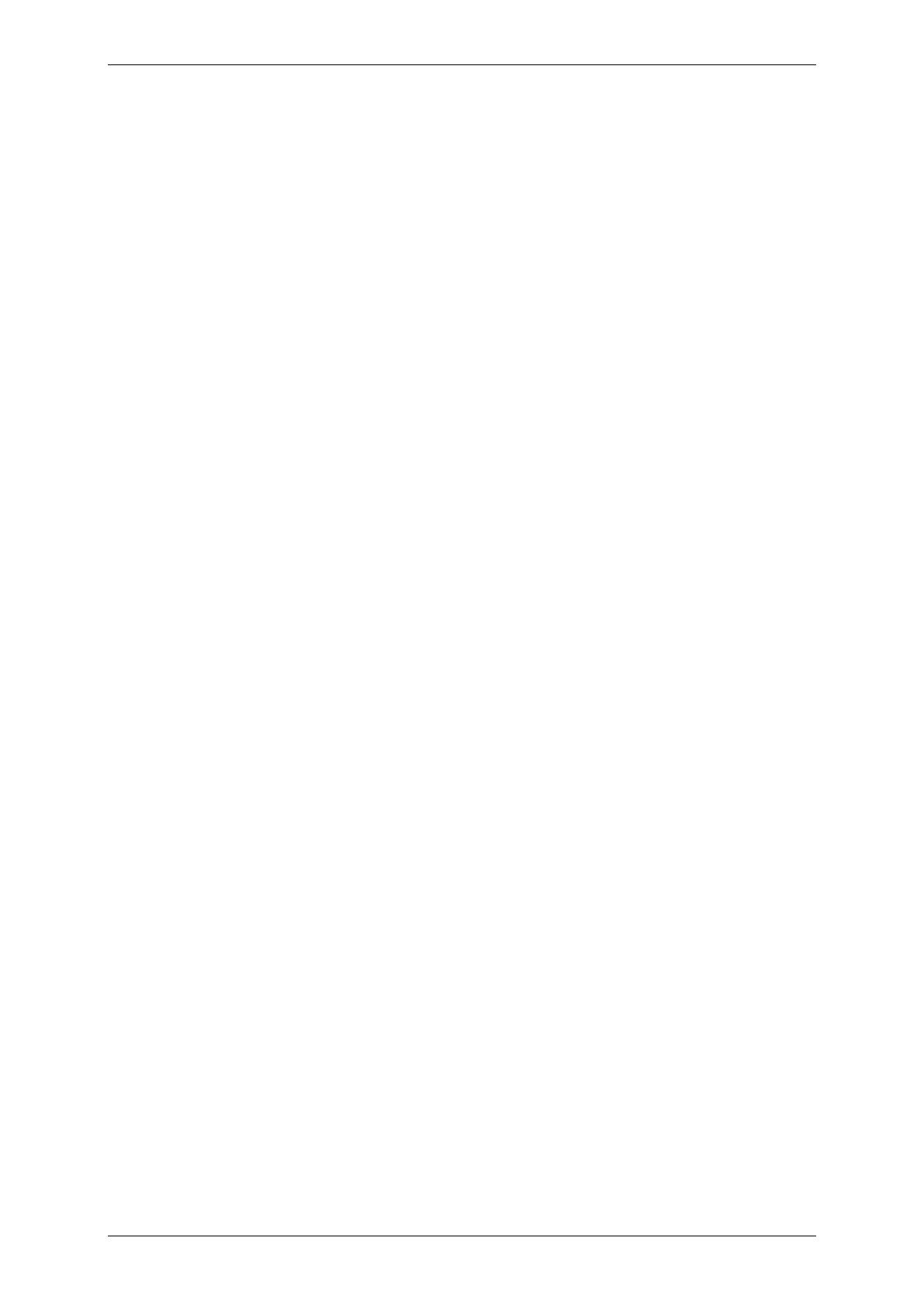
Glossary of terms
OIE Terrestrial Manual 2014 v
• Purity 153
Quality of a biological product prepared to a final form and: 154
a) Relatively free from any extraneous microorganisms and extraneous material (organic or inorganic) as 155 determined by test methods appropriate to the product; and 156
b) Free from extraneous microorganisms or material which could adversely affect the safety, potency or 157 efficacy of the product. 158
• Qualitative Risk Assessment (Handbook on Import Risk Analysis for Animals and Animal 159
Products, Volume 1) 160
An assessment where the outputs of the likelihood of the outcome or the magnitude of the consequences are 161 expressed in qualitative terms such as high, medium, low or negligible. 162
• Quantitative Risk Assessment (Handbook on Import Risk Analysis for Animals and Animal 163
Products, Volume 1) 164
An assessment where the outputs of the of the risk assessment are expressed numerically. 165
• Reference animal 166
Any animal for which the infection status can be defined in unequivocal terms; may include diseased, infected, 167 vaccinated, immunised or naïve animals. 168
• Reference Laboratory 169
Laboratory of recognised scientific and diagnostic expertise for a particular animal disease and/or testing 170 methodology; includes capability for characterising and assigning values to reference reagents and samples. 171
• Repeatability 172
Level of agreement between replicates of a sample both within and between runs of the same test method in a 173 given laboratory. 174
• Reproducibility 175
Ability of a test method to provide consistent results when applied to aliquots of the same sample tested by the 176 same method in different laboratories. 177
• Risk (OIE Handbook on Import Risk Analysis for Animals and Animal Products, Volume 1) 178
The likelihood of the occurrence and the likelihood magnitude of the biological and economic consequences of an 179 adverse event or effect to animal or human health. 180
• Risk Communication (Handbook on Import Risk Analysis for Animals and Animal 181
Products, Volume 1) 182
The interactive transmission and exchange of information and opinions throughout the risk analysis process 183 concerning risk, risk-related factors and risk perceptions among risk assessors, risk managers, risk 184 communicators, the general public, and other interested parties. 185
• Room temperature 186
The term ‘room temperature’ is intended to imply the temperature of a comfortable working environment. Precise 187 limits for this cannot be set, but guiding figures are 18–25°C. Where a test specifies room temperature, this 188 should be achieved, with air conditioning if necessary; otherwise the test parameters may be affected. 189
• Safety 190
Freedom from properties causing undue local or systemic reactions when used as recommended or suggested by 191 the manufacturer and without known hazard to in-contact animals, humans and the environment. 192
• Sample 193
Material that is derived from a specimen and used for testing purposes. 194