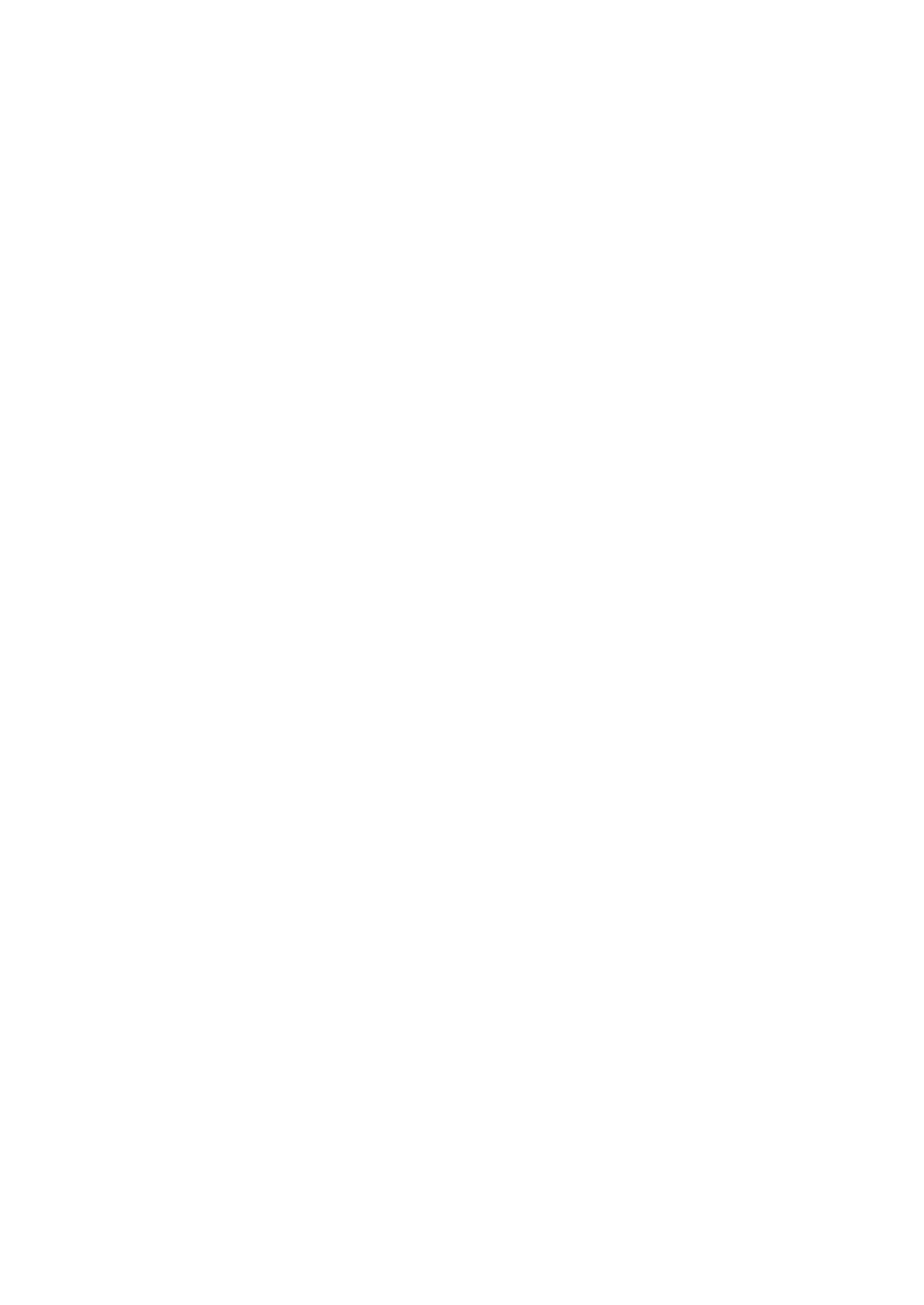
TABLE OF CONTENTS
1 INTRODUCTION ...................................................................................... 14
1.1 Cancer .................................................................................................. 15
1.1.1 Cancer and the Immune System .................................................. 16
1.1.2 Tumor Immunology ....................................................................... 16
1.1.3 Cancer Related Inflammation (CRI) ............................................. 17
1.2 Innate and adaptive immune response to tumors ................................ 18
1.3 Immune cells and anti-cancer therapies ............................................... 21
1.4 Cells in the tumor microenvironment .................................................... 23
1.4.1 Inflammatory cell component of tumors ....................................... 24
1.4.2 Tumor-associated leukocytes ....................................................... 25
1.4.3 Macrophages ................................................................................ 26
1.4.3.1 Tumor associated macrophages .............................................. 26
1.4.3.1.1 TAMs phenotype ................................................................ 27
1.4.4 Monocytes .................................................................................... 32
1.4.4.1 Monocyte heterogeneity ........................................................... 33
1.4.4.2 Monocytes sub populations ...................................................... 35
1.4.4.3 Recruitment of monocytes to sites of inflammation ................. 38
1.4.4.4 Ly6Chigh and Ly6Clow monocytes .............................................. 39
1.4.4.5 Monocytes differentiation to macrophages .............................. 42
1.4.4.6 Monocytes recruitment to tumors ............................................. 43
1.5 Monocytes generation .......................................................................... 46
1.6 Animal models ...................................................................................... 48
1.7 Objectives ............................................................................................. 48
2 MATERIALS AND METHODS.................................................................. 49
2.1 Mouse strains ....................................................................................... 50
2.2 Cell lines and culture ............................................................................ 50
2.2.1 MDA-MB-231 cell line ................................................................... 50
2.2.2 C26 cell line .................................................................................. 51