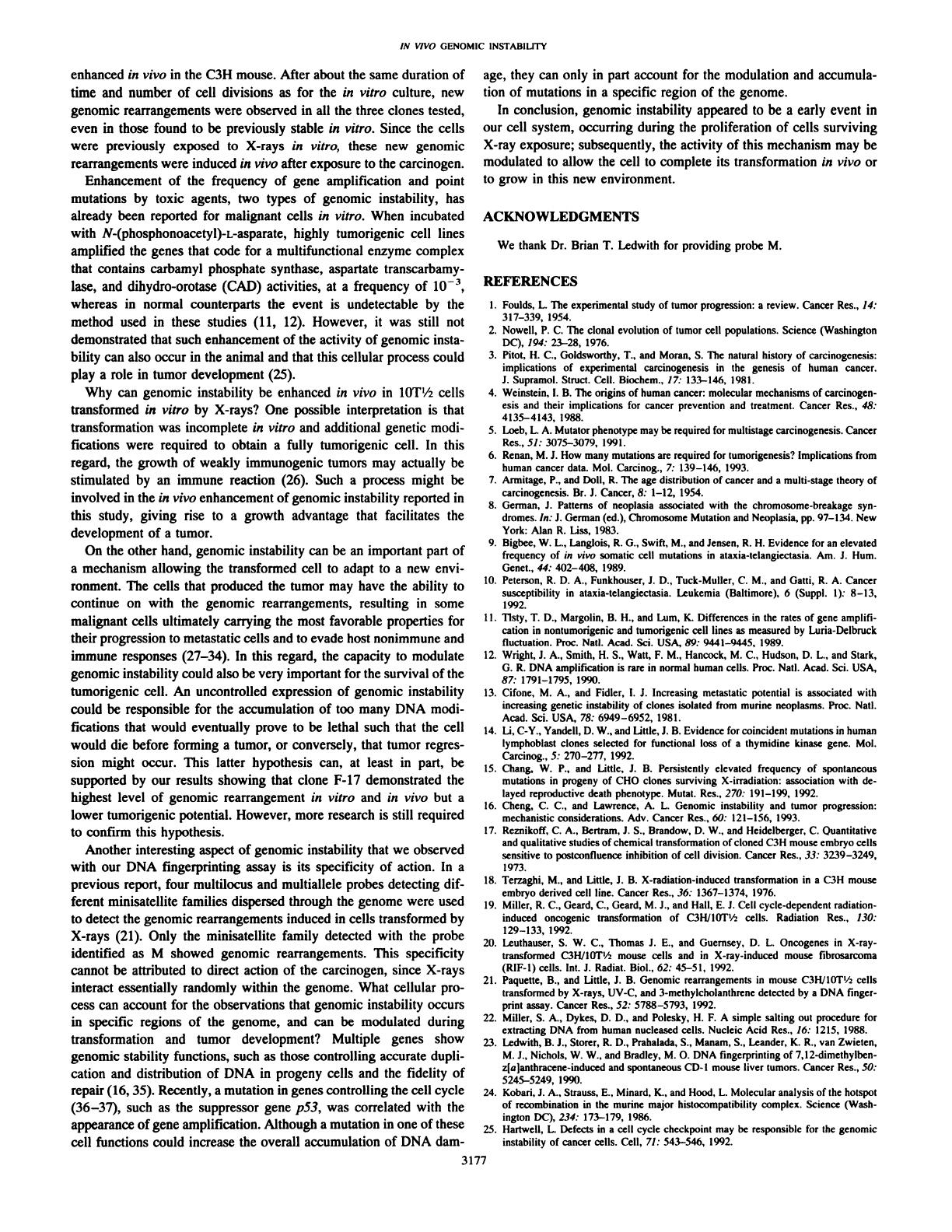
IN VIVO GENOMIC INSTABILITY
enhanced in vivo in the C3H mouse. After about the same duration of
time and number of cell divisions as for the in vitro culture, new
genomic rearrangements were observed in all the three clones tested,
even in those found to be previously stable in vitro. Since the cells
were previously exposed to X-rays in vitro, these new genomic
rearrangements were induced in vivo after exposure to the carcinogen.
Enhancement of the frequency of gene amplification and point
mutations by toxic agents, two types of genomic instability, has
already been reported for malignant cells in vitro. When incubated
with N-(phosphonoacetyl)-L-asparate, highly tumorigenic cell lines
amplified the genes that code for a multifunctional enzyme complex
that contains carbamyl phosphate synthase, aspartate transcarbamy
lase, and dihydro-orotase (CAD) activities, at a frequency of iO@,
whereas in normal counterparts the event is undetectable by the
method used in these studies (ii, 12). However, it was still not
demonstrated that such enhancement of the activity of genomic insta
biity can also occur in the animal and that this cellular process could
play a role in tumor development (25).
Why can genomic instability be enhanced in vivo in 10T½cells
transformed in vitro by X-rays? One possible interpretation is that
transformation was incomplete in vitro and additional genetic modi
fications were required to obtain a fully tumorigenic cell. In this
regard, the growth of weakly immunogenic tumors may actually be
stimulated by an immune reaction (26). Such a process might be
involved in the in vivo enhancement of genomic instability reported in
this study, giving rise to a growth advantage that facilitates the
development of a tumor.
On the other hand, genomic instability can be an important part of
a mechanism allowing the transformed cell to adapt to a new envi
ronment. The cells that produced the tumor may have the ability to
continue on with the genomic rearrangements, resulting in some
malignant cells ultimately carrying the most favorable properties for
their progression to metastatic cells and to evade host nonimmune and
immune responses (21—34).In this regard, the capacity to modulate
genomic instability could also be very important for the survival of the
tumorigenic cell. An uncontrolled expression of genomic instability
could be responsible for the accumulation of too many DNA modi
fications that would eventually prove to be lethal such that the cell
would die before forming a tumor, or conversely, that tumor regres
sion might occur. This latter hypothesis can, at least in part, be
supported by our results showing that clone F-il demonstrated the
highest level of genomic rearrangement in vitro and in vivo but a
lower tumorigenic potential. However, more research is still required
to confirm this hypothesis.
Another interesting aspect of genomic instability that we observed
with our DNA fingerprinting assay is its specificity of action. In a
previous report, four multilocus and multiallele probes detecting dif
ferent minisatellite families dispersed through the genome were used
to detect the genomic rearrangements induced in cells transformed by
X-rays (21). Only the minisatellite family detected with the probe
identified as M showed genomic rearrangements. This specificity
cannot be attributed to direct action of the carcinogen, since X-rays
interact essentially randomly within the genome. What cellular pro
cess can account for the observations that genomic instability occurs
in specific regions of the genome, and can be modulated during
transformation and tumor development? Multiple genes show
genomic stability functions, such as those controlling accurate dupli
cation and distribution of DNA in progeny cells and the fidelity of
repair(i6, 35). Recently, a mutation in genes controlling the cell cycle
(36—37), such as the suppressor gene p.53, was correlated with the
appearance of gene amplification. Although a mutation in one of these
cell functions could increase the overall accumulation of DNA dam
age, they can only in part account for the modulation and accumula
tion of mutations in a specific region of the genome.
In conclusion, genomic instability appeared to be a early event in
our cell system, occurring during the proliferation of cells surviving
X-ray exposure; subsequently, the activity of this mechanism may be
modulated to allow the cell to complete its transformation in vivo or
to grow in this new environment.
ACKNOWLEDGMENTS
We thank Dr. Brian T. Ledwith for providing probe M.
REFERENCES
I. Foulds, L. The experimental study of tumor progression: a review. Cancer Res., 14:
317—339,1954.
2. Nowell, P. C. The clonal evolution of tumor cell populations. Science (Washington
DC), 194: 23-28, 1976.
3. Pitot, H. C., Ooldsworthy, T., and Moran, S. The natural history of carcinogenesis:
implications of experimental carcinogenesis in the genesis of human cancer.
J. Supramol. Struct. Cell. Biochem., 17: 133—146,1981.
4. Weinstein, I. B. The origins of human cancer: molecular mechanisms of carcinogen
esis and their implications for cancer prevention and treatment. Cancer Res., 48:
4135—4143, 1988.
5. Loeb, L. A. Mutator phenotype may be required for multistage carcinogenesis. Cancer
Res., 51: 3075—3079,1991.
6. Renan, M. J. How many mutations are required for tumorigenesis? Implications from
human cancer data. Mol. Carcinog., 7: 139—146,1993.
7. Armitage, P., and Doll, R. The age distribution of cancer and a multi-stage theory of
carcinogenesis. Br. J. Cancer, 8: 1—12,1954.
8. German, J. Patterns of neoplasia associated with the chromosome-breakage syn
dromes. in: 1. German (ed), Chromosome Mutation and Neoplasia, pp. 97—134.New
York: Alan R. Liss, 1983.
9. Bigbee, W. L., Langlois, R. G., Swift, M., and Jensen, R. H. Evidence for an elevated
frequency of in vivo somatic cell mutations in ataxia-telangiectasia. Am. J. Hum.
Genet., 44: 402—408,1989.
10. Peterson, R. D. A., Funkhouser, J. D., Tuck-Muller, C. M., and Gatti, R. A. Cancer
susceptibility in ataxia-telangiectasia. Leukemia (Baltimore), 6 (Suppl. I): 8—13,
1992.
11. Tlsty, T. D., Margolin, B. H., and Lum, K. Differences in the rates of gene amplifi
cation in nontumorigenic and tumorigenic cell lines as measured by Luria-Delbruck
fluctuation. Proc. NatI. Acad. Sd. USA, 89: 9441—9445,1989.
12. Wright, J. A., Smith, H. S., Watt, F. M., Hancock, M. C., Hudson, D. L, and Stark,
0. R. DNA amplification is rare in normal human cells. Proc. NatI. Acad. Sci. USA,
87: 1791—1795,1990.
13. Cifone, M. A., and Fidler, I. J. Increasing metastatic potential is associated with
increasing genetic instability of clones isolated from murine neoplasms. Proc. NatI.
Acad. Sci. USA, 78: 6949—6952,1981.
14. Li,C-Y.,Yandell,D.W.,andLittle,J.B.Evidenceforcoincidentmutationsinhuman
lymphoblast clones selected for functional loss of a thymidine kinase gene. Mol.
Carcinog., 5: 270—277,1992.
15. Chang, W'. P., and Little, 1. B. Persistently elevated frequency of spontaneous
mutations in progeny of CHO clones surviving X-irradiation: association with de
layed reproductive death phenotype. Mutat. Res., 270: 191—199,1992.
16. Cheng, C. C., and Lawrence, A. L. Genomic instability and tumor progression:
mechanistic considerations. Adv. Cancer Res., 60: 121—156,1993.
17. Reznikoff, C. A., Bertram, 1. S., Brandow, D. W., and Heidelberger, C. Quantitative
and qualitative studies of chemical transformation of cloned C3H mouse embryo cells
sensitive to postconfluence inhibition of cell division. Cancer Rca., 33: 3239—3249,
1973.
18.Terzaghi,M.,andLittle,J. B. X-radiation-inducedtransformationin a C3Hmouse
embryo derived cell line. Cancer Rca., 36: 1367—1374,1976.
19. Miller, R. C., Geard, C., Geard, M. J., and Hall, E. J. Cell cycle-dependent radiation
induced oncogenic transformation of C3H/lOT½cells. Radiation Res., 130:
129—133,1992.
20. Leuthauser, S. W. C., Thomas J. E., and Guernsey, D. L. Oncogenes in X-ray
transformed C3H/10T½mouse cells and in X-ray-induced mouse fibrosarcoma
(RIF-1) cells. tnt. J. Radial. Biol., 62: 45—51,1992.
21. Paquette, B., and Little, J. B. Genomic rearrangements in mouse C3H/10T½ cells
transformed by X-rays, UV-C, and 3-methylcholanthrene detected by a DNA finger
print assay. Cancer Res., 52: 5788—5793,1992.
22. Miller, S. A., Dykes, D. D., and Polesky, H. F. A simple salting out procedure for
extracting DNA from human nucleased cells. Nucleic Acid Res., 16: 1215, 1988.
23. Ledwith, B. 1., Storer, R. D., Prahalada, S., Manam, S., Leander, K. R., van Zwieten,
M. J., Nichols, W. W., and Bradley, M. 0. DNA fingerprinting of 7,12-dimethylben
z[a]anthracene-induced and spontaneous CD-I mouse liver tumors. Cancer Res., 50:
5245—5249,1990.
24. Kobari, J. A., Strauss, E., Minard, K., and Hood, L. Molecular analysis of the hotspot
of recombination in the murine major histocompatibility complex. Science (Wash
ington DC), 234: 173—179,1986.
25. Hartwell, L. Defects in a cell cycle checkpoint may be responsible for the genomic
instability of cancer cells. Cell, 71: 543—546,1992.
3177
on April 23, 2015. © 1994 American Association for Cancer Research. cancerres.aacrjournals.org Downloaded from