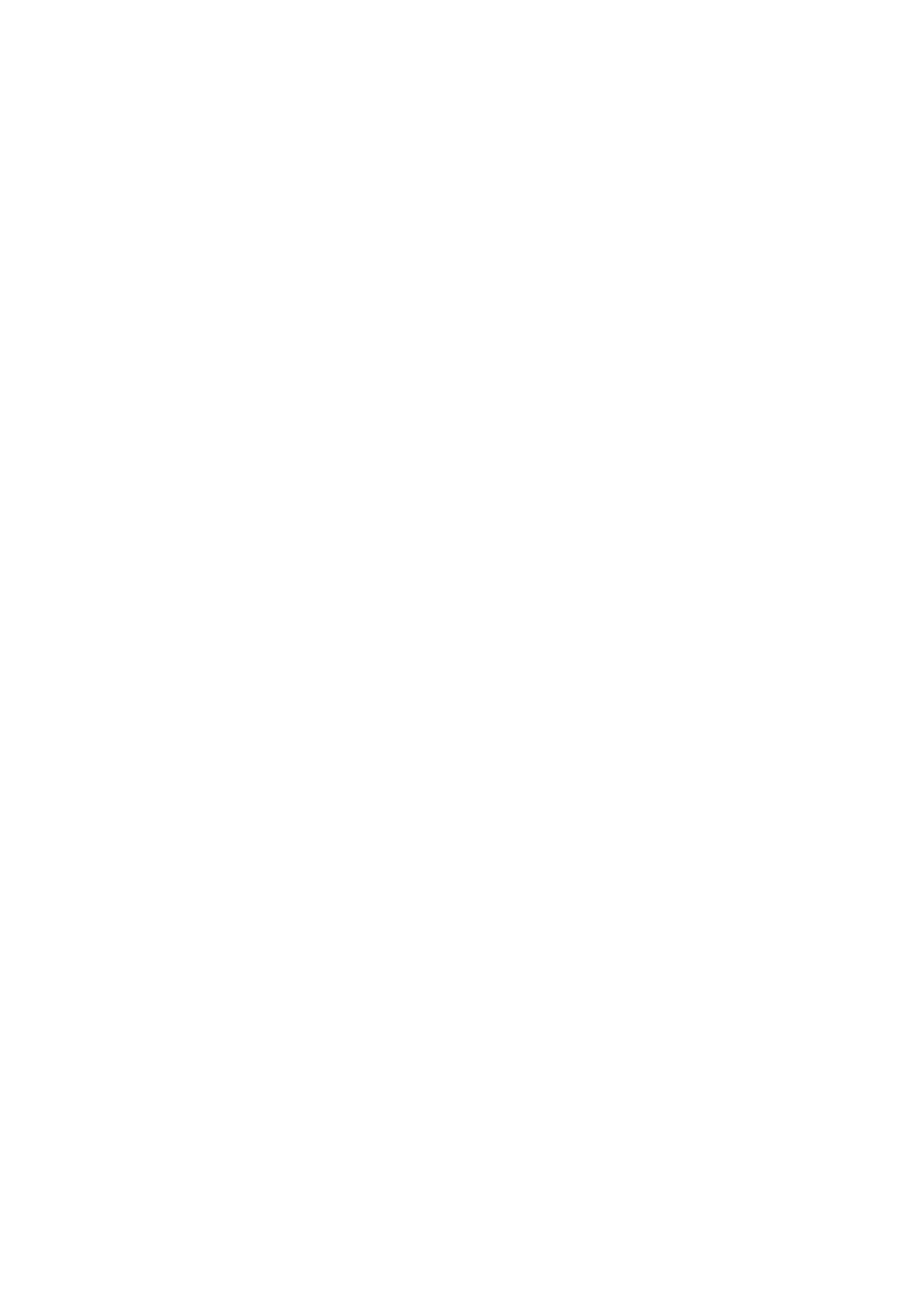
REMERCIEMENTS
Je voudrais tout d’abord remercier les membres de mon jury qui me font l’honneur de juger ce
travail de thèse. Je remercie le Dr. Eve-Isabelle Pêcheur et le Dr. Camille Sureau d’avoir
accepté la charge d’être rapporteur externe de mon travail de thèse. Je remercie de même le
Pr. Sylvie Fournel qui, après avoir jugé mon travail à mi-parcours lors de mon Comité de
thèse, a accepté d’être encore une fois rapporteur interne.
Je voudrais sincèrement remercier le Pr. Thomas Baumert, directeur de l’unité U748 et mon
directeur de thèse. Merci de m’avoir donnée la chance de travailler dans ton équipe. Merci
pour ton encadrement, ton dynamisme, ton soutien.
Merci aussi au Pr. Françoise Stoll-Keller, ma directrice de thèse, qui m’a suivie tout au long
de ma thèse malgré un emploi du temps très chargé. Merci pour tous ces moments passés dans
votre bureau à parler à la fois de travail mais aussi pour m’avoir écoutée pendant mes
moments de doutes.
Je tiens à remercier profondément le Dr. Jean-Pierre Martin. Merci Jean-Pierre de m’avoir
encadrée durant mon stage de M2. Merci de m’avoir appris toutes ces techniques qui m’ont
permises d’avancer dans ma thèse. Et surtout merci de ne m’avoir jamais abandonnée, malgré
le fait que tu partais à la retraire, et de m’avoir mise en de bonnes mains pour ma thèse !
Voilà quatre années qui viennent de s’écouler. Le travail fut intense et cela n’aurait pas été
aussi simple si je n’avais pas été entourée de tant de collègues et d’amis.
Merci à Samira. Merci de m’avoir toujours encouragée dans mon travail. Merci pour toutes
ces discussions, à la fois sur le travail, mais aussi sur « tout et rien », qui m’ont permises
parfois de décompresser quand les journées devenaient longues.
Merci à ma chère Michèle. Michèle, ces quatre années n’auraient pas été les mêmes si je ne
les avais pas passées en ta présence. Tu as toujours été là quand je ne savais plus vers qui me
tourner. Merci pour ton aide au travail. Merci pour, pareil, toutes nos discussions (et il y en a
eu!).
Merci à Mirjam. Merci pour ta disponibilité dans le travail. Merci pour ton aide lorsque je
cherchais des réponses à mes questions. Merci pour ta bonne humeur constante. Merci tout
simplement pour tout.
Merci à mon voisin de bureau Joachim. On en a passé des bons moments! Entre les cours de
français que je te donnais, ta façon de toujours étaler toutes tes affaires sur mon bureau. Merci
pour ton sourire tous les matins quand j’arrivais au labo.
Merci à Marine, Laetitia, Alizé, Karine, Daniel, Nauman, Patric, Valentin, Marina, Richard,
Julien, Géraldine, Thomas pour tous ces moments de fou-rires à la fois à la cafeteria (avec les
mots croisés), sur la terrasse pour une petite pause, et dans le bureau des thésards. Soyez
toujours là les uns pour les autres, vous êtes vraiment géniaux !