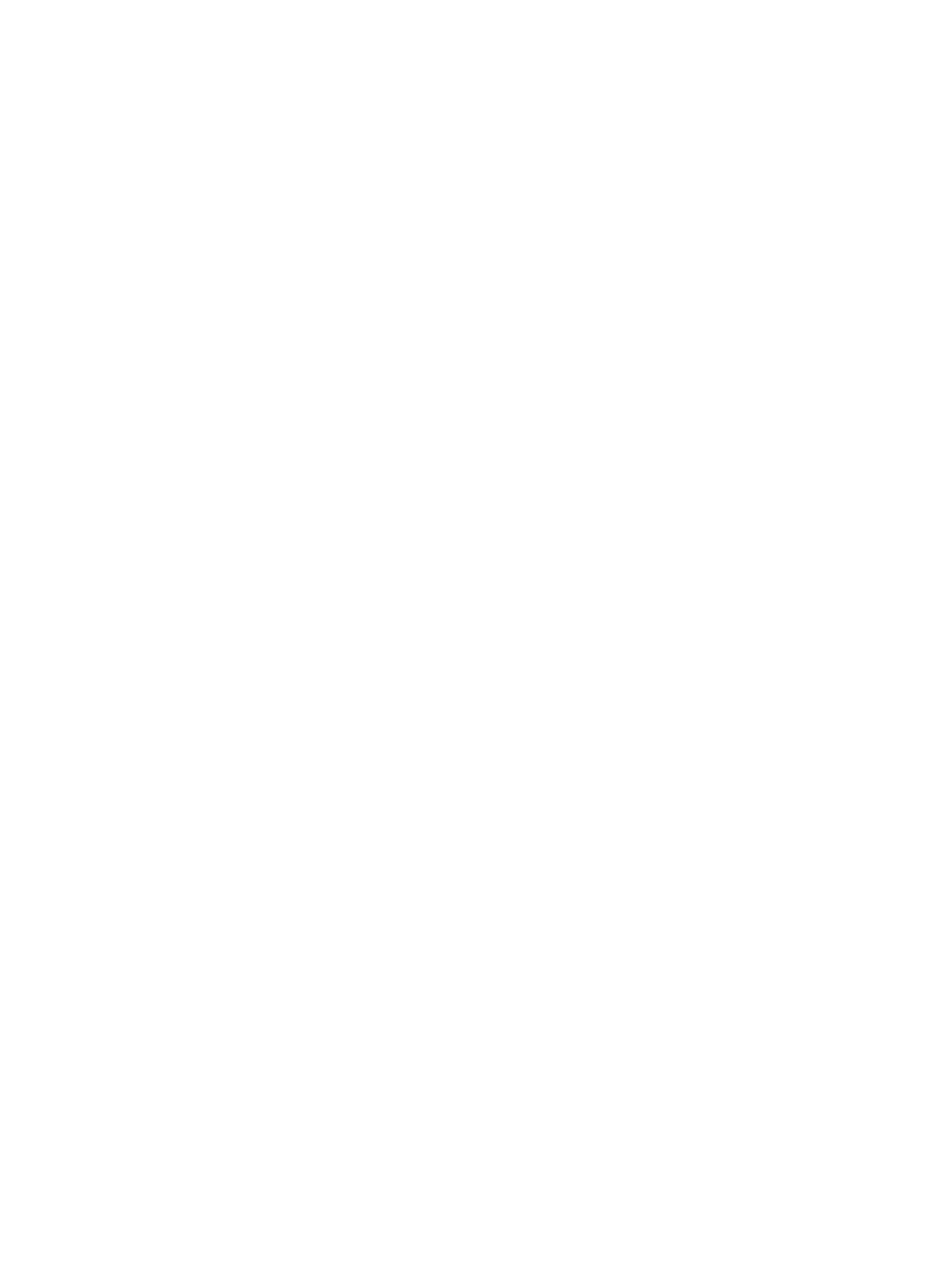
Le Bail et al.,1993).Consistentwiththesedata,thelower
growthrateafterGHimmunodepletionprovedtheneedfor
endogenousGHinnormalgrowth(LeBailet al., 1991). In
addition,IGF1injectionincreasesshgrowth(McCormick
et al.,1992;Chenet al.,2000)andendogenousplasmaIGF1
levelsareoftencorrelatedwithgrowthrate(Beckmanand
Dickhoff,1998;Pierceet al.,2001;Mingarroet al.,2002).
AlltheseobservationsraisethepossibilitythattheGH/IGF
systemcanmediatetheeffectsoftemperatureongrowthand
development.
AfterashortdescriptionoftheGH/IGFsysteminsh,
wewillpresentthecurrentknowledgeconcerningtheeffect
oftemperatureontheGH/IGFsystemduringtheembryonic
andpost-larvalperiods.Hypotheses,explainingtheeffects
oftemperatureonGH/IGFsystemactivity,willalsobedis-
cussed.
ThegeneralorganisationoftheGH/IGFsystemissimi-
larinbothhighervertebratesandsh,andincludesgrowth
hormone(GH),GHreceptor,IGF1,IGF2,IGFreceptors,
andIGFbindingproteins(Plisetskayaet al.,1994;Duan,
1997;Kelleyet al.,2000).
Growthhormoneissynthesizedinthepars distalisofthe
pituitaryandwaspuriedinshforthersttimeintilapia
(Oreochromis mossambica)(Farmeret al.,1976).Itsexpres-
sionandsecretiondependonseveralneuropeptides(GHRH,
NPY,SRIF,GnRH,dopamine,etc.)andhormones(T3,
IGF1,IGF2,etc.)(HollowayandLeatherland,1998;Peng
andPeter,1997;PeterandChang,1999).Incontrasttomam-
mals,endogenousbasalsecretionofGHinshisveryhigh
in vitro (Yada et al.,1991;YadaandHirano,1992;Blaiseet
al.,1995a),givingtheinhibitorsofGHsecretionagreater
impact(SRIF,IGFs,etc.).
TheGHreceptor,belongingtothecytokinereceptor
family,hasbeenmainlycharacterizedbybindingstudies,in
severalshspecies(YaoandLeBail,1999;Hirano,1991;
Gray et al.,1992;Nget al.,1992;Yaoet al.,1991;Zhang
andMarchant,1996).RecentlycDNAfortheGHreceptorin
goldsh(Carassius auratus) (Lee et al.,2001),turbot(Pset-
ta maxima)(Calduch-Gineret al.,2001)andseabream
(Sparus aurata)(Calduch-Gineret al.,2003)werecloned
andsequenced,revealingthewell-conservedmotifcharac-
teristicsoftheGHreceptor.Asobservedinmammals,the
liveristhemainorganexpressingtheGHreceptor(Yaoand
LeBail,1999;Yaoet al.,1991;Hirano,1991;Marti-Palanca
andPérez-Sánchez,1994;Calduch-Gineret al.,2001).
IGFs(IGF1andIGF2)arehighlyconservedbetweensh
andmammals(>70%similarity),underlyingtheimportance
ofthesegrowthfactorsthroughoutevolution(Chenet al.,
1994).Consistentwiththe‘Somatomedin’hypothesis,exog-
enousGHstimulatesIGF1expressionintheshliver(Cao
et al.,1989;Shamblottet al.,1995;Moriyama,1995;Dug-
uay et al.,1996;Guillénet al.,1998),whichisthemajor
sourceofcirculatingIGF1(Leroithet al.,2001).Inrainbow
trout(Oncorhynchus mykiss)butnotinothersspecies,GH
canstimulatehepaticIGF2expressionin vivo and in vitro
(Shamblottet al.,1995;LeBailet al.,1998).AlthoughIGF1
functionappearstobelinkedtogrowthregulation(Chenet
al.,1994;BeckmanandDickhoff,1998;Beckmanet al.,
2001;Mingarroet al.,2002),thespecicroleofIGF2isnot
yetknown.Recently,Gabillardet al.(2003c)reportedthat
plasmaIGF2levelsareassociatedwiththenutritionalstatus
ratherthanwiththegrowthrate.
TheIGFtypeIreceptormediatesthebiologicalactions
oftheIGFs.Thisreceptorisaheterotetramer,withtwoextra-
cellularαsubunitsandtwointracellularβsubunits.Theα
subunitcontainstheligand-bindingdomainandtheβ sub-
unitbearsthetyrosinekinasedomainimplicatedinthesig-
naltransduction.Bindingcharacteristicshavebeenstudied
inseveralshspeciesandtheamino-acidsequencesindicate
awell-conservedstructureinteleosts(Navarroet al.,1999;
Planas et al.,2000).Inmammals,asecondIGFreceptor
bindsIGF2:themannose-6-phosphate/IGF2receptor(M6P/
IGF2receptor).Inamphibianandbirdsthisreceptordoes
notbindIGF2duetoalackofaspecicbindingsite(Can-
fieldandKornfeld,1989;Kiesset al.,1994).Therecent
reportofanIGF2bindingtothisreceptorinrainbowtrout
(Mendezet al.,2001)raisesquestionsaboutitsevolution
andfunction.
Asinmammals,IGFscirculateinbloodboundtospecif-
icbindingproteins(IGFBP).Inseveralshspecies,three
formsofIGFBParepresentinblood(Kelleyet al.,2000;
Kelley et al.,2002)withapparentmolecularmassesof29
kDa(IGFBP1),31kDa(IGFBP2)and40-43kDa(IGFBP3).
Theirnucleotidesequencesarenowavailableinzebrash
(Danio rerio) (Duan et al.,1999;MauresandDuan,2002),
seabream(Funkensteinet al.,2002),tilapia(Chenget al.,
2002)andturbot(Duval,2000)anddataconcerningthe
affinityforIGF1andIGF2aswellastheirregulationhas
beguntobereported(Kajimuraet al.,2003;Shimizuet al.,
2003).
Infish,biologicalfunctionsandexternalfactorsinflu-
encetheGH/IGFsystemactivity.Forinstance,salmonsmol-
tificationleadstoanincreaseinplasmalevelsofGHand
IGF1beforetransfertoseawater(Bœuf,1987a;Bœuf,
1987b;Dickhoffet al.,1997).Inaddition,duringsexual
maturationofseveralshspecies,plasmalevelsofGHand
IGF1increase,leadingtoseasonalvariationsofhormonal
Does the GH/IGF system mediate the effect of water temperature on sh growth? Gabillard e t a l .
108 Cybium 2005, 29(2)