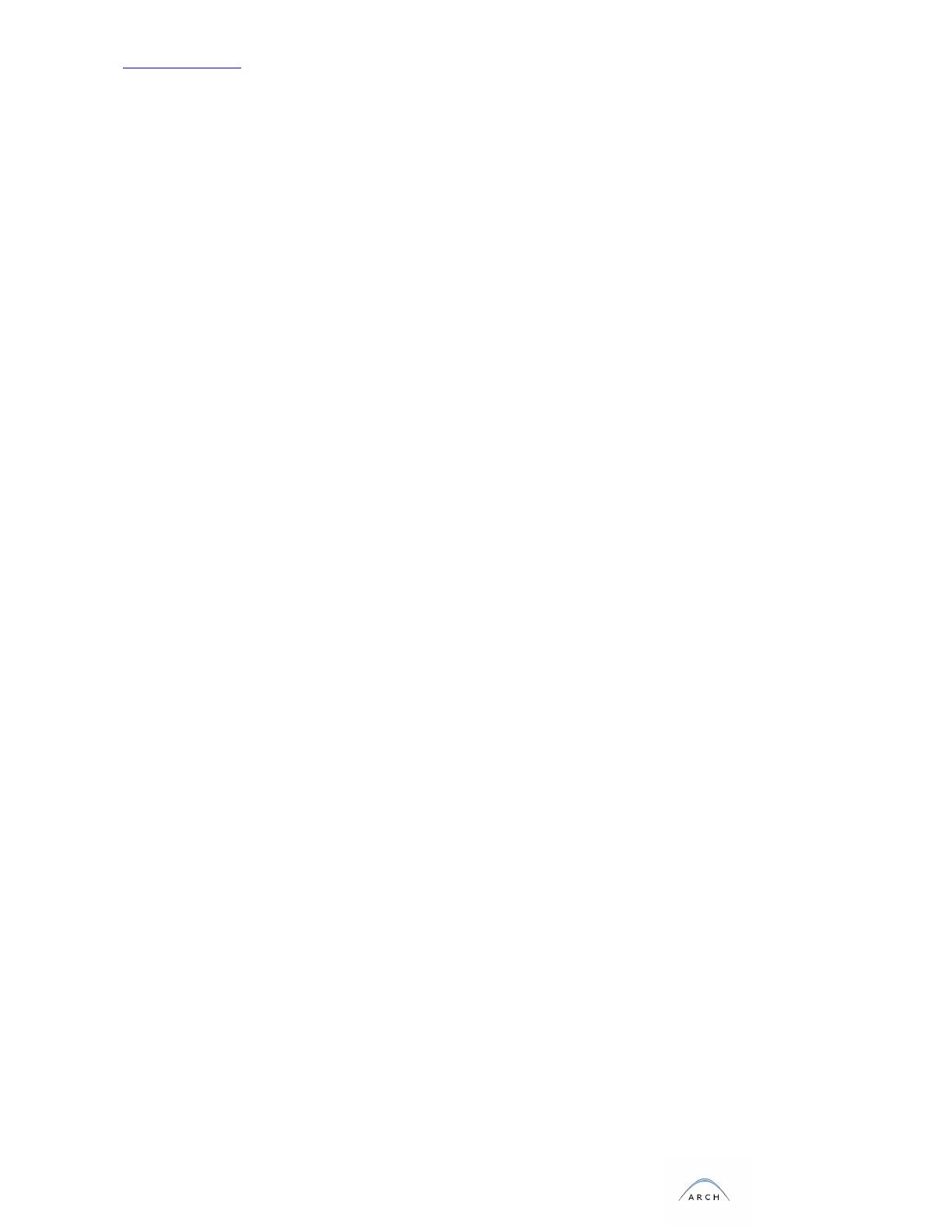
3
Doses.................................................................................................................................................. 28
Biological samples.............................................................................................................................. 28
Data collection .................................................................................................................................... 28
Molecular markers .............................................................................................................................. 28
Feasibility............................................................................................................................................ 28
Proposed approaches - Objective 2........................................................................................................ 28
Study Population................................................................................................................................. 29
Study Design ...................................................................................................................................... 29
Doses.................................................................................................................................................. 29
Data collection .................................................................................................................................... 29
Biological samples.............................................................................................................................. 29
Molecular markers .............................................................................................................................. 29
Feasibility............................................................................................................................................ 29
Proposed approaches - Objective 3........................................................................................................ 30
Study Population................................................................................................................................. 30
Study Design ...................................................................................................................................... 30
Data collection .................................................................................................................................... 30
Biological samples.............................................................................................................................. 30
Feasibility............................................................................................................................................ 30
Proposed approaches - Objective 4........................................................................................................ 30
Study Population................................................................................................................................. 30
Study Design ...................................................................................................................................... 31
Doses.................................................................................................................................................. 31
Data collection .................................................................................................................................... 31
Biological samples.............................................................................................................................. 31
Molecular markers .............................................................................................................................. 31
Feasibility............................................................................................................................................ 31
Proposed approaches - Objective 5........................................................................................................ 31
Study Population................................................................................................................................. 31
Study Design ...................................................................................................................................... 31
Doses.................................................................................................................................................. 32
Biological samples.............................................................................................................................. 32
Data collection .................................................................................................................................... 32
Molecular markers .............................................................................................................................. 32
Feasibility............................................................................................................................................ 32
Prioritisation ............................................................................................................................................ 32
LEUKAEMIA AND LYMPHOMA....................................................................................................33
Background............................................................................................................................................. 33
General ............................................................................................................................................... 33
Studies of leukaemia and lymphoma in the post-Chernobyl period................................................... 34
In utero exposure ........................................................................................................................... 34
Childhood exposure ....................................................................................................................... 35
Adulthood exposure ....................................................................................................................... 36
Clean-up workers ........................................................................................................................... 36
Objectives ............................................................................................................................................... 37
Specific relevance (value-addedness) of Chernobyl populations........................................................... 37
Proposed approach – Objective 1........................................................................................................... 37
Population and study design .............................................................................................................. 37
Dosimetry............................................................................................................................................ 38
Biological samples.............................................................................................................................. 38
Molecular markers .............................................................................................................................. 38
Pathology............................................................................................................................................ 38
Feasibility - Roadblocks that need to be overcome............................................................................ 38
Ethics requirements............................................................................................................................ 38
Statistical power.................................................................................................................................. 38
Prioritisation........................................................................................................................................ 38
Proposed approach - Objective 2 ........................................................................................................... 39
Population and study design .............................................................................................................. 39
Doses.................................................................................................................................................. 39
Biological samples.............................................................................................................................. 39
Molecular markers.......................................................................................................................... 39
Pathology ....................................................................................................................................... 39