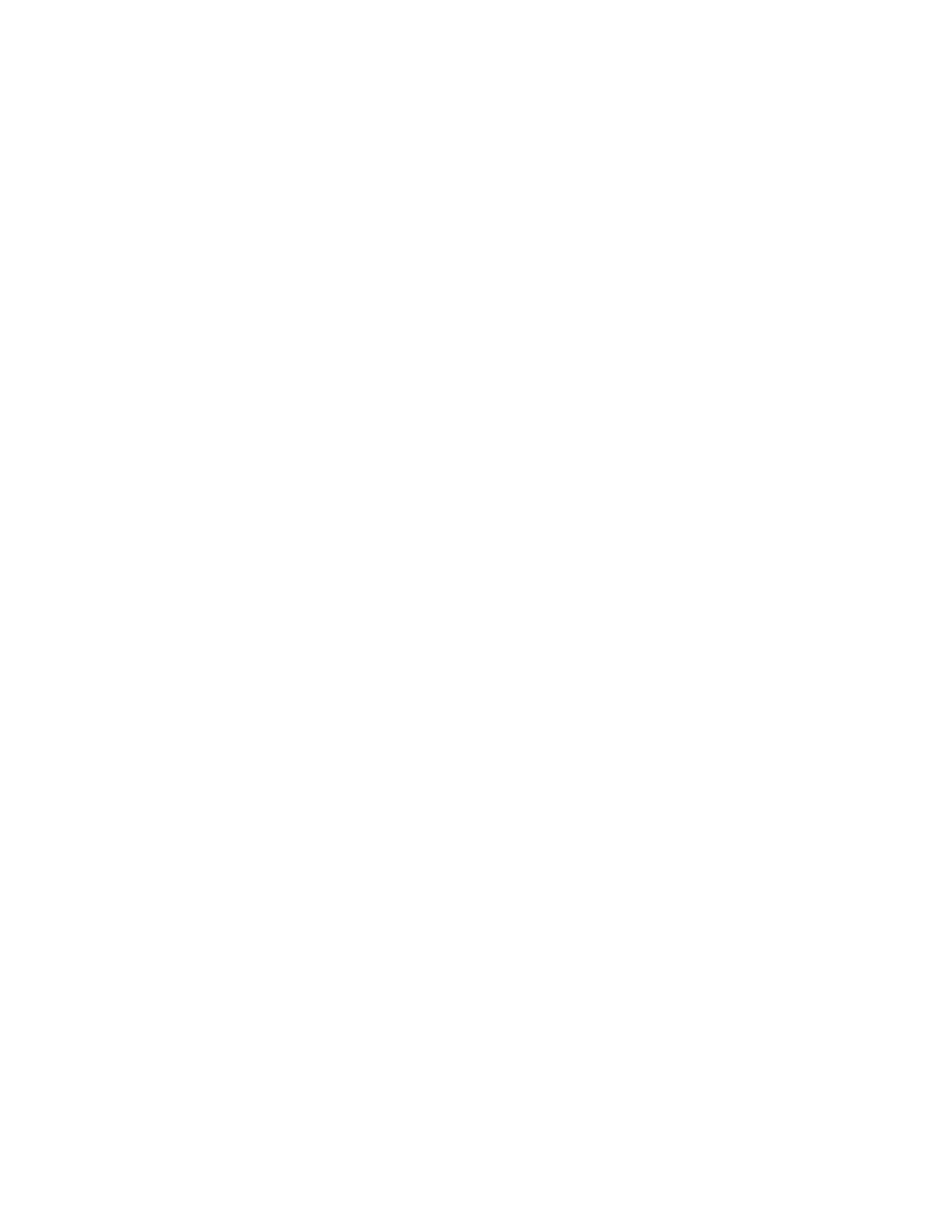
v
Abstract
In Canada, peat moss is extracted for production of horticultural substrate on an industrial scale. Several
studies have contributed to develop restoration techniques for open Sphagnum-dominated habitats on the peat
massif of peatlands. Beside tree plantation trials, however, peatland margins and their connectivity with
surrounding landscapes has received little attention. The goal of this thesis is to acquire the ecological
knowledge required to develop an ecological restoration strategy adapted to the bog - mineral land (upland)
forest transition (aka the “lagg” habitat of the peatland). A first study of the bog-ecotone-forest gradient showed
that the lagg is a true ecotone, with unique properties: 1) vegetation is more structured, with a dense shrubby
stratum (Ilex mucronata often present); 2) nitrogen and phosphorus soil concentrations are high; 3) water table
level fluctuates widely, but remains high for relatively long periods. A second study aimed at improving our
knowledge of the baseline ecological conditions in cutaway peat fields located within the marginal areas of
extracted peatlands. One major result is that soil nutrient concentrations in cutaway peat fields are very low:
for phosphorus and potassium, approximately one order of magnitude lower when compared to natural laggs.
Also, in exception to recolonization in former ditches, there is little spontaneous recolonization. In this same
study, one area rehabilitated through afforestation (20-year-old coniferous plantations) was also characterized
to compare its ecological status to unrestored fields: results suggest that afforestation ameliorates abiotic
micro-site environmental conditions (richer soils and more temperate soil-air microclimate), but spontaneous
recolonization does not occur. As reintroduction seems inevitable, two studies were conducted to improve our
ecological knowledge of ecological filters in cutaway areas, and to assess the establishment potentials of
some target species: one study on bryophytes, and one study on shrubs. Among the reintroduced species, two
showed higher establishment potential: the bryophyte Dicranum polysetum and the shrub Ilex (Nemopanthus)
mucronata. Shade significantly ameliorated the establishment potential of the reintroduced bryophytes
Sphagnum and Dicranum, and of the shrub Ilex. Fertilization significantly increased Ilex seedling growth, while
presence of a Dicranum carpet significantly increased seedling survival through a reduction of frost-heaving
effects. In conclusion, the combination of exploratory and experimental studies allowed us to significantly
improve our knowledge of bog - mineral land forest transitions, and to suggest guidelines for an ecological
restoration strategy leading to a functioning lagg ecotone.