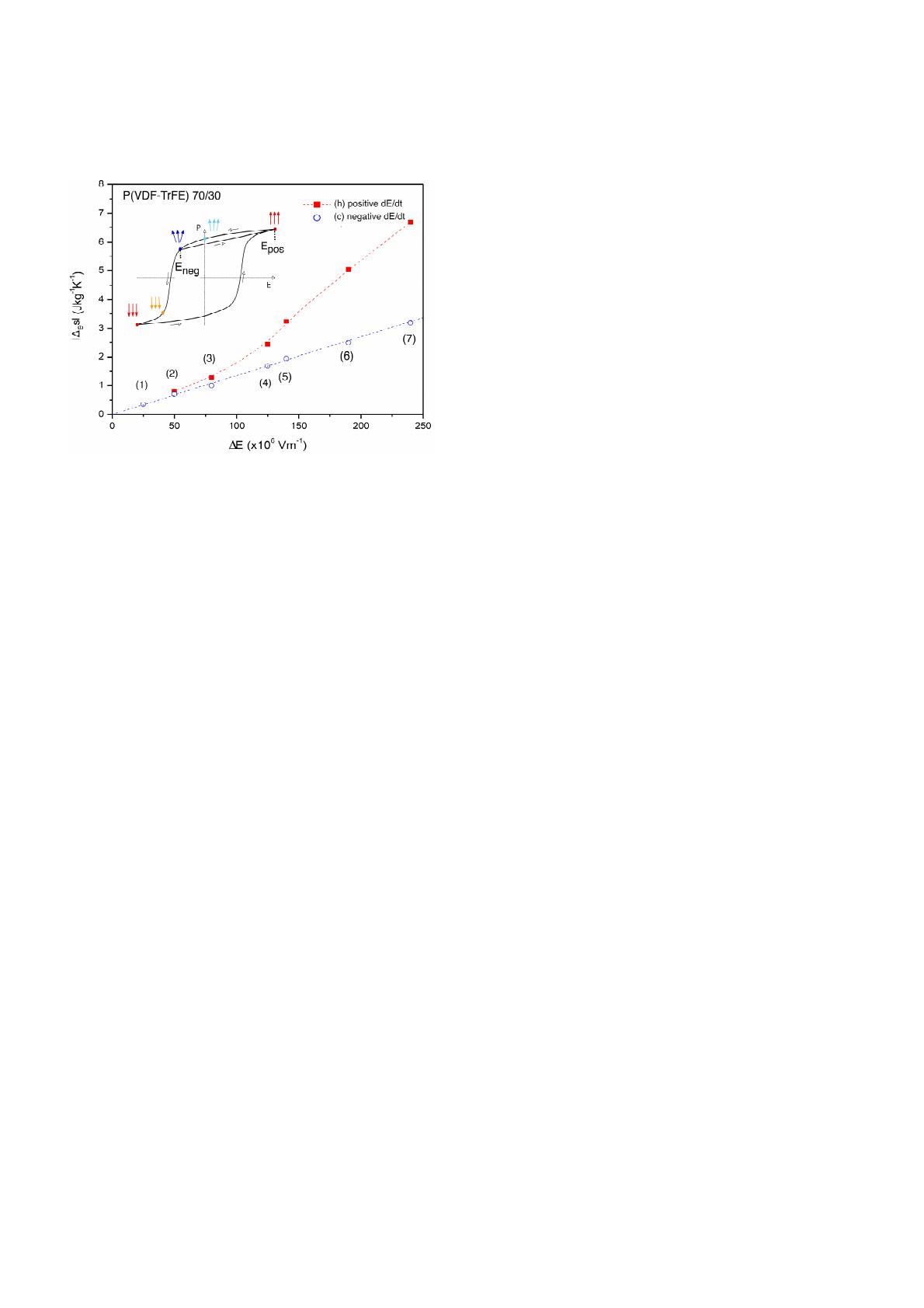
(-40/40) ou (0/80) aboutissent à la même variation
d’entropie réversible mais, pour le premier cycle, le champ
électrique maximum appliqué étant plus petit, les pertes
par effet Joule générées par la conduction électrique du
matériau s’en trouvent réduites.
Figure 2.Variation d’entropie autour de la polarisation
rémanente du P(VDF-TrFE) (70/30) obtenue pour des
cycles asymétriques. Insert : schéma d’un cycles P(E)
symétrique et asymétrique. Variation d’entropie
change │∆eS│en function de │∆E│= Epos - Eneg, with
(Eneg, Epos): (1) (0,25); (2) (-25, 25); (3) (-40, 40); (4) (-
25, 100); (5) (-40, 100); (6) (-40,150); (7) (-40, 200) (x
106Vm-1).
Conclusion
En conclusion, un dispositif avec des modules Peltier a été
développé pour réaliser une mesure directe de l’effet
électrocalorique. Ce dispositif permet également de séparer
les contributions réversibles (effet électrocalorique) et
irréversibles (pertes hystérétiques), paramètre important
pour concevoir un système de refroidissement
électrocalorique. Les cycles asymétriques semblent être
intéressants pour améliorer les performances
électrocaloriques des matériaux sans augmenter le champ
électrique maximum appliqué. Ainsi, l’impact de la
conductivité électrique du matériau sur l’efficacité de
conversion électrique-thermique s’en trouve réduit.
L’efficacité d’un matériau électrocalorique est le point clé
dans le développement de systèmes de froid [12].
Références
1. Mischenko, A.S., Q. Zhang, J.F. Scott, R.W.
Whatmore, and N.D. Mathur, Giant
electrocaloric effect in thin-film PbZr0.95Ti0.05O3.
Science, 2006. 311: p. 270-271.
2. Neese, B., B. Chu, S.G. Lu, Y. Wang, E. Furman,
and Q.M. Zhang, Large electrocaloric effect in
ferroelectric polymers near room temperature.
Science, 2008. 321: p. 821-823.
3. Lu, S.G., B. Rozic, Q.M. Zhang, Z. Kutnjak, R.
Pirc, M. Lin, X. Li, and L. Gorny, Comparison of
directly and indirectly measured electrocaloric
effect in relaxor ferroelectric polymers. Appl.
Phys. Lett., 2010. 97: p. 202901.
4. Kar-Narayan, S. and N.D. Mathur, Direct and
indirect electrocaloric measurements using
multilayer capacitors. J. Phys. D: Appl. Phys,
2010. 43: p. 032002.
5. Lu, S.G., B. Rozic, Q.M. Zhang, Z. Kutnjak, X.
Li, E. Furman, L.J. Gorny, M. Lin, B. Malic, M.
Kosec, R. Blinc, and R. Pirc, Organic and
inorganic relaxor ferroelectrics with giant
electrocaloric effect. Appl. Phys. Lett., 2010. 97:
p. 162904.
6. Sebald, G., L. Seveyrat, J.-F. Capsal, P.-J.
Cottinet, and D. Guyomar, Differential scanning
calorimeter and infrared imaging for
electrocaloric characterization of poly(vinylidene
fluoride-trifluoroethylene-chlorofluoroethylene)
terpolymer. Appl. Phys. Lett., 2012. 101: p.
022907.
7. Sebald, G., S. Pruvost, L. Seveyrat, L. Lebrun, D.
Guyomar, and B. Guiffard, Electrocaloric
properties of high dielectric constant ferroelectric
ceramics. J. Eur. Ceram. Soc., 2007. 27: p. 4021-
4024.
8. Sebald, G., L. Seveyrat, D. Guyomar, L. Lebrun,
B. Guiffard, and S. Pruvost, Electrocaloric and
pyroelectric properties of 0.75Pb(Mg1/3Nb2/3)O3-
0.25PbTiO3 single crystals. J. Appl. Phys., 2006.
100: p. 124112.
9. Bai, Y., G.-P. Zheng, and S.-Q. Shi, Kinetic
electrocaloric effect and giant net cooling of lead-
free ferroelectric refrigerants. J. Appl. Phys.,
2010. 108: p. 104102.
10. Basso, V., F. Russo, J.-F. Gerard, and S. Pruvost,
Direct measurement of the electrocaloric effect in
poly(vinylidene fluoride-trifluoroethylene-
chlorotrifluoroethylene) terpolymer films. Appl.
Phys. Lett., 2013. 103: p. 202904.
11. Basso, V., J.-F. Gerard, and S. Pruvost, Doubling
the electrocaloric cooling of poled ferroelectric
materials by bipolar cycling. Appl. Phys. Lett.,
2014. 105: p. 052907.
12. Defay, E., S. Crossley, S. Kar-Narayan, X. Moya,
and N.D. Mathur, The electrocaloric efficiency of
ceramic and polymer films. Adv. Mater., 2013.
25(24): p. 3337-3342.