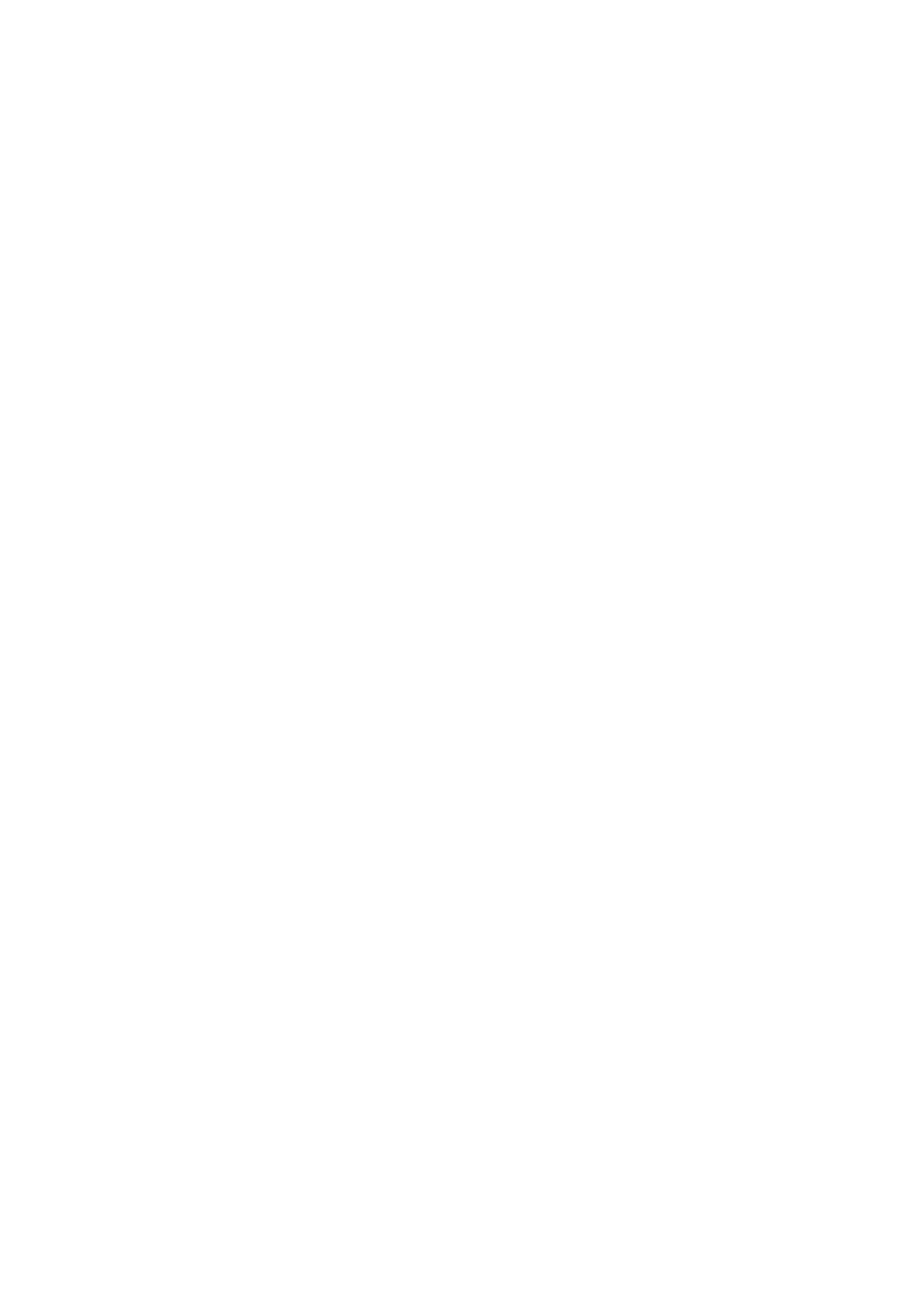
V
SHORT SUMMARY
Breast cancer (BC) is the second leading cause of cancer death in western women. Its
incidence continues to increase, especially in post-menopausal women. Exploring the
pathogenesis and seeking new treatments remain hotspots. In spite of the increasing
number of studies on 17β-hydroxysteroid dehydrogenases (17β-HSDs), the expression
and role of 17β-HSD5 (AKR1C3) remain controversial. Here we answered the question
whether 17β-HSD5 is a possible target for BC therapy and made the comparison of
AKR1C3 expression in normal breast and tumor samples. In addition, we propose that
the lower expression of AKR1C3 is a biomarker for poor prognostic in breast cancer.
We suggest to provide DHEA as intracrinological hormone source and to compare the
role of steroid-converting enzymes using DHEA and their direct substrates. We
demonstrated that provision of DHEA was a good choice to mimic postmenopausal
condition in steroid metabolism in cell culture.