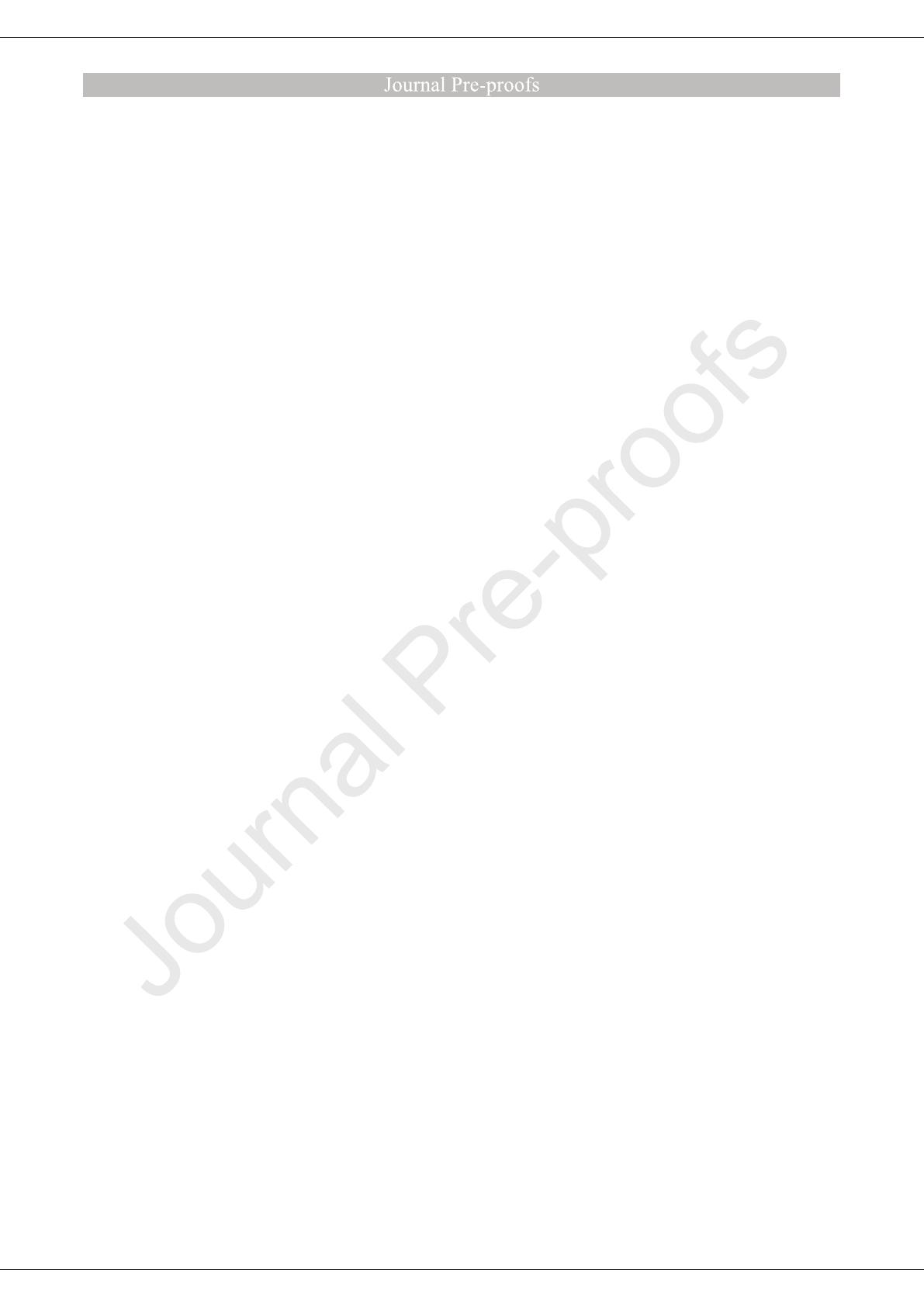
1. Introduction
Oily sludge is mainly generated during the production, refining, storage, and
transportation of petroleum (Deng et al., 2016; Liang et al., 2017), and includes mud
from the drilling process, waste oil in the well, emulsified solids created during the
crude oil refining process, and sediment in the storage tank (Fig. 1) (Deng et al., 2015;
Vivana et al., 2015; Wang et al., 2017). In China, the annual sludge production is close
to 5 million tons (Gong et al., 2018; Wang et al., 2019). With the continuous growth of
the oil industry, the development of unconventional oil and gas fields has continued to
increase (Santos et al., 2014), s has the production of oily sludge (Fig. 2) (BP, 2019).
Oily sludge is generally a complex emulsifying mixture composed of water,
heteroatoms (N, O, S), heavy metals (Ca, V, Fe, Ni), crude oil, solid particles, and
various surfactants (Wang et al., 2018a; Castaneda et al., 2014). The chemical
compositions and properties of oily sludge vary greatly due to numerous factors such
as the oilfield type, soil composition, and storage conditions; thus, the physicochemical
properties of oily sludge from different sources are not the same (Wang et al., 2019).
The components of crude oil mainly include aliphatic hydrocarbons, aromatic
hydrocarbons, asphaltenes, resins, etc., among which benzene, xylene, cycloalkanes,
polycyclic aromatic hydrocarbons, and other volatile and refractory organic compounds
are relatively common (Hu et al., 2013; Rudyk et al., 2018; Huang et al., 2014a); this
is also one of the reasons why oily sludge is highly viscous, toxic, and acidic, and why
its common form is highly stable oil-in-water (W/O) or water-in-oil (O/W) emulsions
(Langevin et al., 2015; Filho et al., 2012). Therefore, oily sludge poses a serious threat