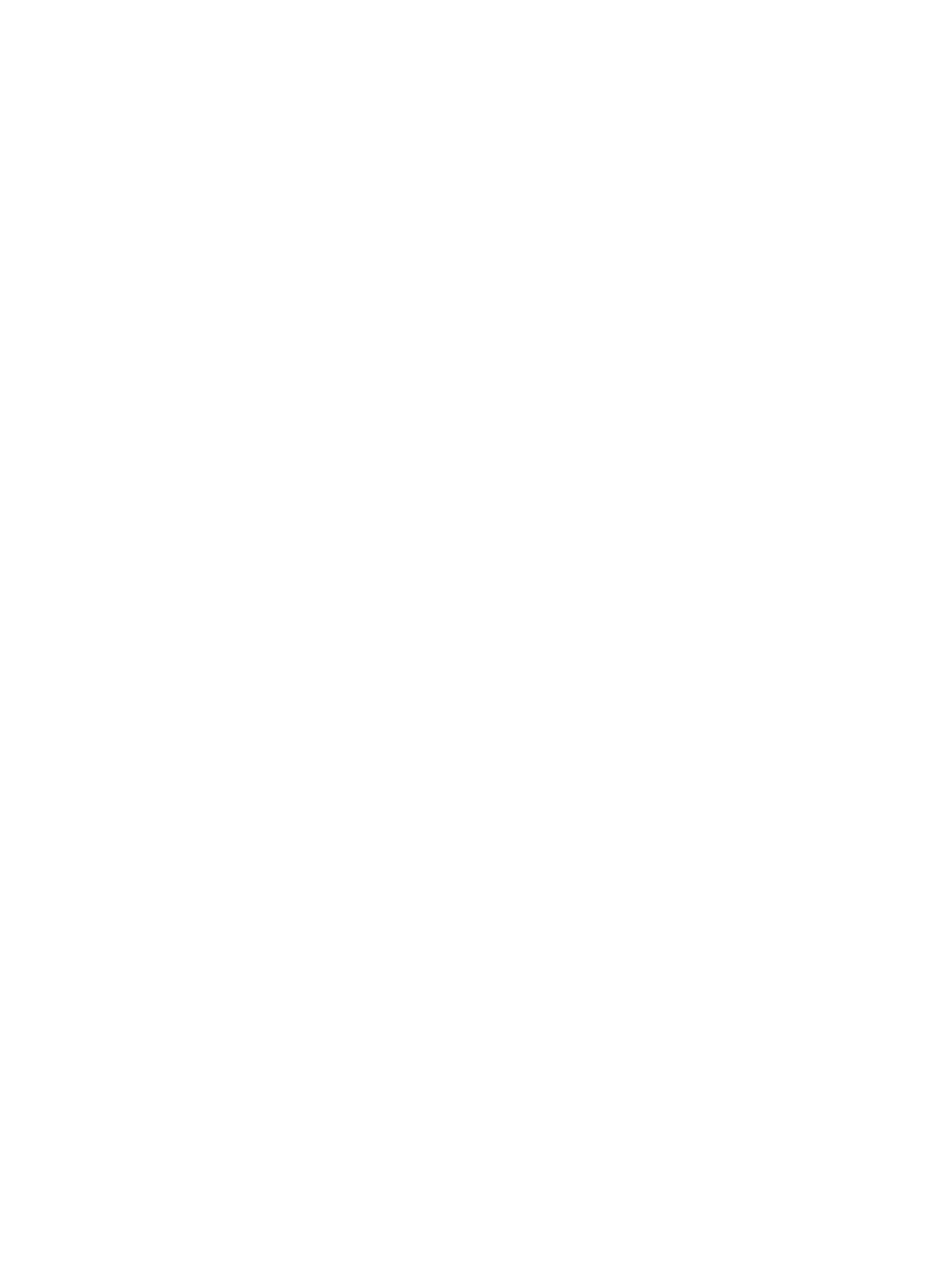
Journal of Functional Foods 90 (2022) 104954
2
cellular processes in both physiological conditions and pathological
stress insults (Fanta et al., 2021). Mitochondrial impairment can induce
hepatocyte death and associated abnormal cellular events, which is a
key factor for NAFLD development and hepatic dysfunction (Eduardo
et al., 2021; Sanda et al., 2021). Emerging evidence has suggested that
defects in mitochondrial hemostasis are common characteristics of fatty
liver and maintaining healthy mitochondria is pivotal for the survival of
hepatocytes, contributing to NAFLD treatment (Lee, Park, & Roh, 2019).
Therefore, methods to suppress oxidative stress and mitochondrial
dysfunction may be effective for NAFLD management.
Recently, endoplasmic reticulum (ER) stress has attracted increasing
attention. ER is a crucial organelle where secreted and transmembrane
proteins are synthesized and correctly folded into three-dimensional
conformations. These above dynamic events need highly precise mod-
ulation to sustain normal protein homeostasis (Marciniak & Ron, 2006;
Schr¨
oder, 2008). Numerous stimuli including HFD can break the above
equilibrium, subsequently inducing the unfolded protein response
(UPR) and ER stress occurrence (Ghemrawi, Battaglia-Hsu, & Arnold,
2018; Iurlaro & Mu˜
noz-Pinedo, 2016). Upon ER stress initiation, GRP78,
as a key ER stress sensor, is released. Severe ER stress can facilitate the
expression of pro-apoptotic proteins, such as CHOP. Finally, cells un-
dergo apoptotic cell death under stimulated conditions (Lee, 2005). In
addition, ER stress may result in the activation of multiple different
intracellular stress pathways, which can induce or accelerate insulin
resistance, dyslipidemia, inammation and, in some cases, culminate in
hepatocyte cell death. All these processes are involved in the patho-
genesis of fatty liver (Zhang et al., 2014). Herein, nding therapeutic
approaches to reduce ER stress may be effective for NAFLD treatment.
Fisetin (FisT; 3,3′,4′,7-tetrahydroxyavone) is an important dietary
avonoid and presents in numerous fruits and vegetables. FisT has
antioxidant, anti-inammatory, anti-cancer and neuroprotective prop-
erties (Grynkiewicz & Demchuk, 2019; Khan et al., 2013; Zhang et al.,
2018). We previously showed that FisT could ameliorate HFD-induced
fatty liver in mice through suppressing hepatic inammation and lipid
deposition by depressing TNF-
α
/RIPK3 signaling pathway (Xu, Ge, &
Qin et al., 2019; Xu, Sun, & Dai et al., 2019). Recently, FisT was
demonstrated to prohibit ROS/ER stress-mediated inammatory
response, contributing to the amelioration of metabolic stimuli-induced
cardiac injury both in vivo and in vitro (Ge et al., 2019). Besides, FisT can
ameliorate oxidative stress, inammation and apoptosis in HFD- or
streptozotocin (STZ)-induced diabetic cardiomyopathy, exerting car-
dioprotective action (Althunibat et al., 2019; Hu et al., 2020). Moreover,
the potential of FisT to regulate mitochondrial function and apoptosis
was shown to suppress tumor growth and protect against myocardial
ischemia–reperfusion (I/R) injury (Pal et al., 2013; Sabarwal, Agarwal,
& Singh, 2017; Shanmugam et al., 2021). Although these protective
effects of FisT against metabolic stresses-triggered liver and heart injury
have been reported, the underlying molecular mechanisms and partic-
ularly the possible crosstalk between them are still not fully understood.
Here in the present study, we performed in vitro and in vivo experi-
ments to deeply explore and conrm the regulatory role of FisT on PA-
and HFD-induced hepatic injury, respectively. We newly found that FisT
could restrain cellular and mitochondrial ROS generation, contributing
to the inhibition of inammatory response and lipid accumulation in PA-
incubated hepatocytes. More importantly, all these effects mediated by
FisT were largely attributed to the suppression of GRP78-mediated ER
stress. The function of FisT to depress ER stress was validated in HFD-
challenged mice, consequently ameliorating NAFLD progression.
2. Materials and methods
2.1. Cells and culture
Human hepatocyte cell line L02 and murine hepatocyte AML12 were
purchased from the Type Culture Collection of the Chinese Academy of
Sciences (Shanghai, China) and American Type Culture Collection
(ATCC; Manassas, VA, USA), respectively. All cells were cultured in
Dulbecco’s Modied Eagle Medium (DMEM) (#22320030, Gibco®)
supplemented with 10% fetal bovine serum (FBS; #10100147, Gibco®)
and 1% penicillin-streptomycin in a humidied incubator (Thermo
Fisher Scientic; USA) with 5% CO
2
at 37 ◦C. To imitate the in vivo liver
lipid deposition, cells were incubated with PA (Cat#P9767) obtained
from Sigma-Aldrich (St. Louis, USA).
2.2. Cell viability
Cell viability was measured using a Cell Counting Kit-8 assay kit
according to the manufacturer’s instructions (#C0039, Beyotime,
Shanghai, China). Briey, cells were planted into 96-well plates. After
treatments, 10
μ
L of Cell Counting Kit-8 reagent was added into each
well. After incubation for 4 h at 37 ◦C, the absorbance of each well at
450 nm was measured using a microplate reader (SpectraMax iD3,
Molecular Devices, USA) to examine the number of viable cells.
2.3. Transfection in vitro
Nrf2 si-RNAs (si-Nrf2), GRP78 si-RNAs (si-GRP78) and the corre-
sponding negative control si-RNAs (si-Con) were obtained from Generay
Biotechnology (Shanghai, China). The GRP78 plasmid based on
pcDNA3.1 vector and empty vector (EV) were constructed by GeneChem
Technology (Shanghai, China) using the standard molecular biology
techniques. Transfection for gene knockdown or over-expression was
performed using Lipofectamine 3000 reagent (Invitrogen Life Technol-
ogies, Carlsbad, USA) according to the provider’s instructions.
2.4. Animals and treatments
The animal study protocols were permitted and authorized by the
Institutional Animal Care and Use Committee in College of Bioengi-
neering, Chongqing University (Chongqing, China). The procedures
used in this study were in accordance with the Regulations of Experi-
mental Animal Administration issued by the Ministry of Science and
Technology of the People’s Republic of China. All male, C57BL/6N mice
(6- to 8-week-old; 22–25 g body weight) used in the study were pur-
chased from Beijing Vital River Laboratory Animal Technology Co., Ltd.
(Beijing, China). Mice were allowed to adapt to their living environment
for 1 week before all experiment proper starts. All animals were fed in a
constant temperature (23 ±25 ◦C), humidity (50–60%) and pathogen-
free-controlled environment cage with a standard 12 h light/12 h dark
cycle, plenty of water and food (pathogen-free) in their cages.
Animal experimental design 1: Mice were then randomly divided
into 4 groups, including (i) the normal chow diet/Vehicle group (NCD/
Veh); (ii) NCD/FisT group; (iii) HFD/Veh group; and (iv) HFD/FisT
group. At the beginning of the experiments, HFD (60 kcal% fat; D12492;
Research Diets, New Brunswick, USA) was subjected to experimental
mice to induce NAFLD murine model. Mice fed with NCD (10.2% fat and
71.5% carbohydrates; D12450B; Research Diets) were served as the
normal controls. For pharmacological analysis of FisT, FisT (80 mg/kg)
as drug (HPLC purity ≥98%, Xi’an Ruiying Biotechnology Co. Ltd,
Xi’an, China) was given to mice by gavage daily with HFD for 16 weeks,
continuously (Xu, Ge, & Qin et al., 2019; Xu, Sun, & Dai et al., 2019).
Vehicle group of mice received the same volume of saline. Body weights
were measured during HFD and FisT treatments weekly.
Animal experimental design 2: Male C57BL/6N mice (6–8 weeks
old; 22–25 g) were fed HFD (D12492, Research Diets) for 16 weeks to
induce NASH. And after 8 weeks of HFD, mice were randomly assigned
to four experimental groups: (i) NCD/Veh; (ii) NCD/FisT group; (iii)
HFD/Veh group; and (iv) HFD/FisT group. FisT (80 mg/kg) was
administrated to mice every day by gavage for 8 weeks. Vehicle group of
mice received the same volume of saline.
After 16 weeks, blood was obtained through retro-orbital bleeding,
and the serum was separated. Liver samples from each mouse were
X. Dai et al.