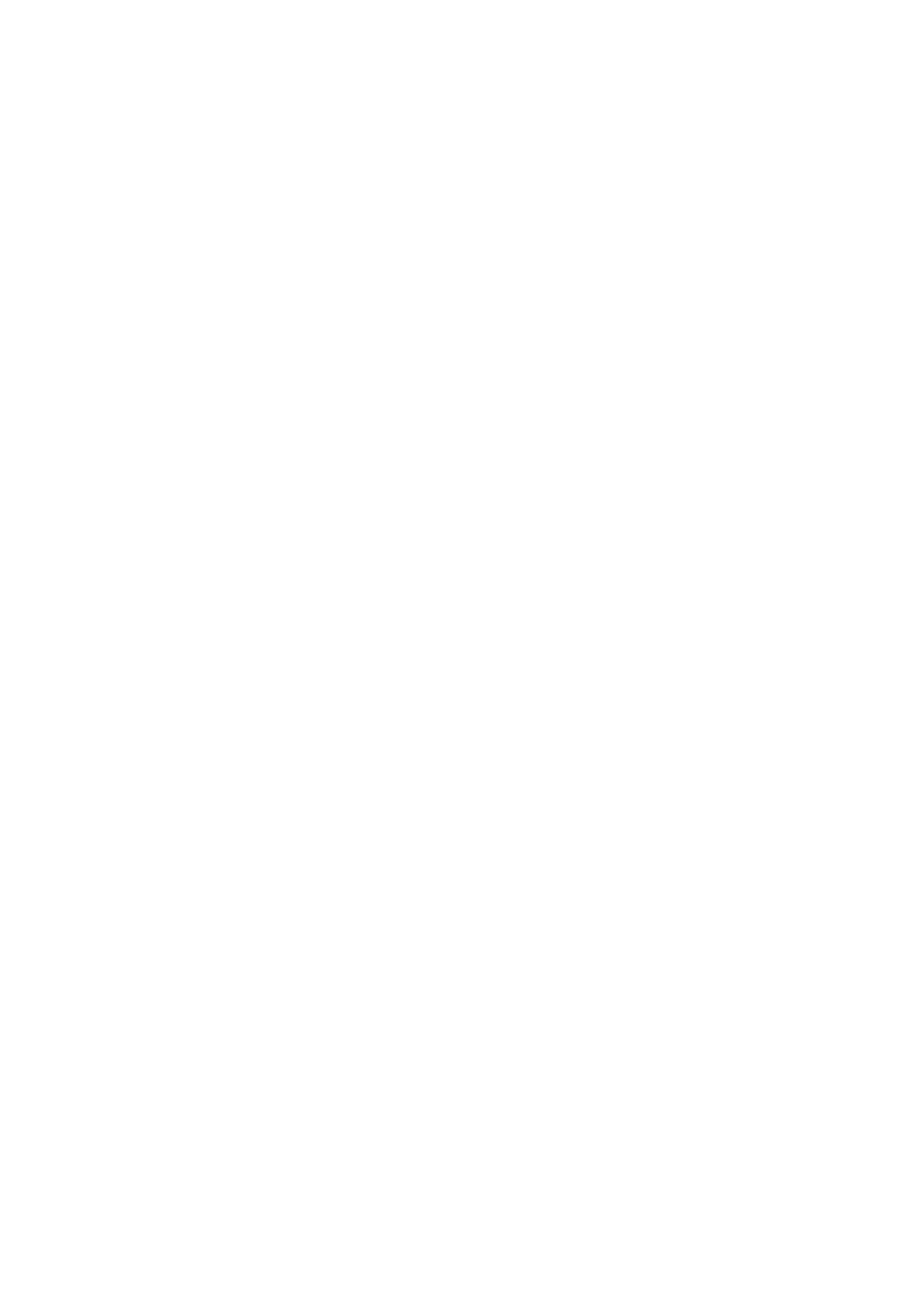
(Burrell, Model 75) overnight until a yellowish brown, vis-
cous solution was obtained. A peristaltic pump was used
to dispense the polymer solution. At the end of the dispen-
sing tube, a pipette tip (Bioplas, Fisher; ID 0.30 mm) was
attached and positioned approximately 7 cm above the
surface of the cation solution used for gel formation. The
peristaltic pump was then programmed to dispense a drop
of polymer solution approximately every 3 s into 1 l of
0.1 M cation solution (Ca, Cu or Fe chloride solution) in
a beaker. A paddle stirrer unit (Phipps and Bird, Model
7790-400) was used to provide gentle stirring (60 rpm)
when forming the gel beads, since the beads were reported
to be susceptible to hydrodynamic forces (Ogbonna et al.,
1991). The acid resistant polypropylene paddles (Cole-
Parmer) were used as paddle assemblies for all mixing and
bead washing.
The beads were allowed to cure in cation solutions for
3 days and then removed from the cation solution and
rinsed twice (either with Milli Q water for Ca and Cu
beads or with 1 mM HCl for Fe and Ca±Fe beads). The
beads were then transferred to a continuous washing sys-
tem (015 ml/min) with in-line acid neutralizing unit. For
preparation of Ca±Fe beads, washed Ca beads were trans-
ferred to 0.100 M FeCl
3
solution for further doping of the
beads with Fe(III). The beads were continuously washed
for 3 days and stored in 1 mM HCl until used (up to
6 months) for As(V) sorption studies.
To con®rm that the bead synthesis was reproducible,
the size distribution of the beads was analyzed. Digitized
photographs of the beads were used in combination with
image analysis software (Optimetric, Optimas), which
automatically counts and measures the diameter of each
bead and calculates size distribution.
Conditions and apparatus for batch sorption experiments
For the batch experiments, 85 ml polycarbonate centri-
fuge tubes were used as reactors with 50 ml of solution as
reaction volume. All of the batch experiments were done
at pH 4 using acetate buer (0.5 mM) and background
electrolyte of 5 mM NaNO
3
unless otherwise speci®ed.
The pH of the solution was adjusted with either HNO
3
(0.1 N) or NaOH (0.1 N) solution, and the pH was
measured with an Orion 720A pH meter. For each repli-
cate in the batch experiments, 5 beads were equilibrated
(unless otherwise speci®ed) in acetate buer solution at
speci®ed pH for a day and transferred to the reaction bot-
tles after the surface moisture of the beads was wiped o.
Then, As(V) spikes (to ®nal concentrations of 1.00 mg/l to
10.0 mg/l) were added. The bottles were placed in a rack,
which was mounted on a wrist action shaker for mixing.
After the speci®ed reaction times, the beads were removed
from the bottles to stop further reaction of arsenate with
the beads, and the supernatants and/or gel beads were
analyzed for As(V). All experiments were run in duplicate.
Analytical measurements
The total Fe in the beads was measured by disrupting
the gel phase by sonication in an EDTA solution (Murata
et al., 1993). The beads were transferred to a 50 ml volu-
metric ¯ask, 3 ml of 0.1 M EDTA was added and the ¯ask
and contents were sonicated (Branson B-220, Smithkline)
for 30 min. The ¯ask was then ®lled to volume with Milli
Q water and ®ltered using 0.1 mm cellulose nitrate mem-
brane ®lter (Sartorius).
Fe analysis by Inductively Coupled Plasma Mass
Spectrometry (ICP-MS, Perkin-Elmer/Sciex Elan 5000A)
was done by measuring the 57Fe isotope. The aqueous
samples were introduced at a rate of 1 ml/min from a
Gilson 212B autosampler and a peristaltic pump to a
cross-¯ow pneumatic nebulizer in a room temperature
spray chamber. High purity Ni skimmer and sampling
cones were used. Calibration standards were run in the
same matrix (by disintegrating Ca beads with EDTA) to
verify that matrix eects did not interfere with ICP-MS
analysis.
Both the initial As(V) and the dissolved As(V) concen-
tration in the solution for each batch experiment were
measured with ICP-MS for low initial As(V) concen-
trations (i.e., 1.00 to 400 mg/l) at 75As without ®ltration.
For initial As(V) concentrations higher than 400 mg/l,
As(V) in the gel phase was measured by the method used
for total Fe analysis in the beads with appropriate cali-
bration standards. All samples were measured with ICP-
MS in 1% HNO
3
solution.
RESULTS AND DISCUSSION
Eects of cation on As(V) sorption
Initially, four gel bead types, Ca, Cu, Fe, and
mixed Ca±Fe beads, were produced and tested for
As(V) sorption. Among the beads tested, Ca±Fe
beads showed highest As(V) removal at 24 h, and
Fe and Ca±Fe beads showed comparable As(V)
removal at 2 h (Fig. 2). Neither Cu beads nor Ca
beads showed any signi®cant As(V) sorption even
after 24 h. Even at an As(V) concentration of 5 mg/
l, the maximum uptake of As(V) by Ca beads was
0.013(20.001) mg per g of wet Ca bead compared
with 1.57(20.03) mg per g of wet Ca±Fe beads.
This absence of As(V) uptake by Ca beads demon-
strates that As(V) accumulation in the pore volume
of Fe and Ca±Fe beads by passive diusion is negli-
gible compared with speci®cally bound As(V). This
is consistent with the negligibly small water volume
in the beads as compared with the total solution
volume in the batch experiments. With Cu beads,
the poor As(V) removal may be attributable to the
formation of an impervious layer at the surface of
the Cu beads which inhibits diusion of solutes into
the beads as suggested by previous studies
(Martinsen et al., 1989; Velings and Mestdagh,
1995).
Although Fe beads were easier to produce than
Ca±Fe beads, the physical integrity of the Fe beads
was poor with extreme cracking and peeling at the
surface. The mixed Ca±Fe beads, which were in-
itially formed with Ca and then doped with Fe,
showed good mechanical stability. In the Ca±Fe
beads, Ca may act as primary gel forming cation
providing a stable structure for the polymer net-
work. The Fe then partly replaces the Ca in the gel
matrix to provide favorable sorption sites for
As(V). The average measured Fe content of a single
Ca±Fe bead was 25.0 20.5 mg (0.4520.01 mmol).
All the subsequent experiments were performed
with the Ca±Fe beads.
As(V) sorption kinetics and eects of initial As(V)
concentration
Partitioning of As(V) between the solution and
gel phase at pH 4 is shown as a function of time in
Fig. 3. Excellent mass balance was obtained
throughout the experiment by summing the
Joon H. Min and Janet G. Hering1546