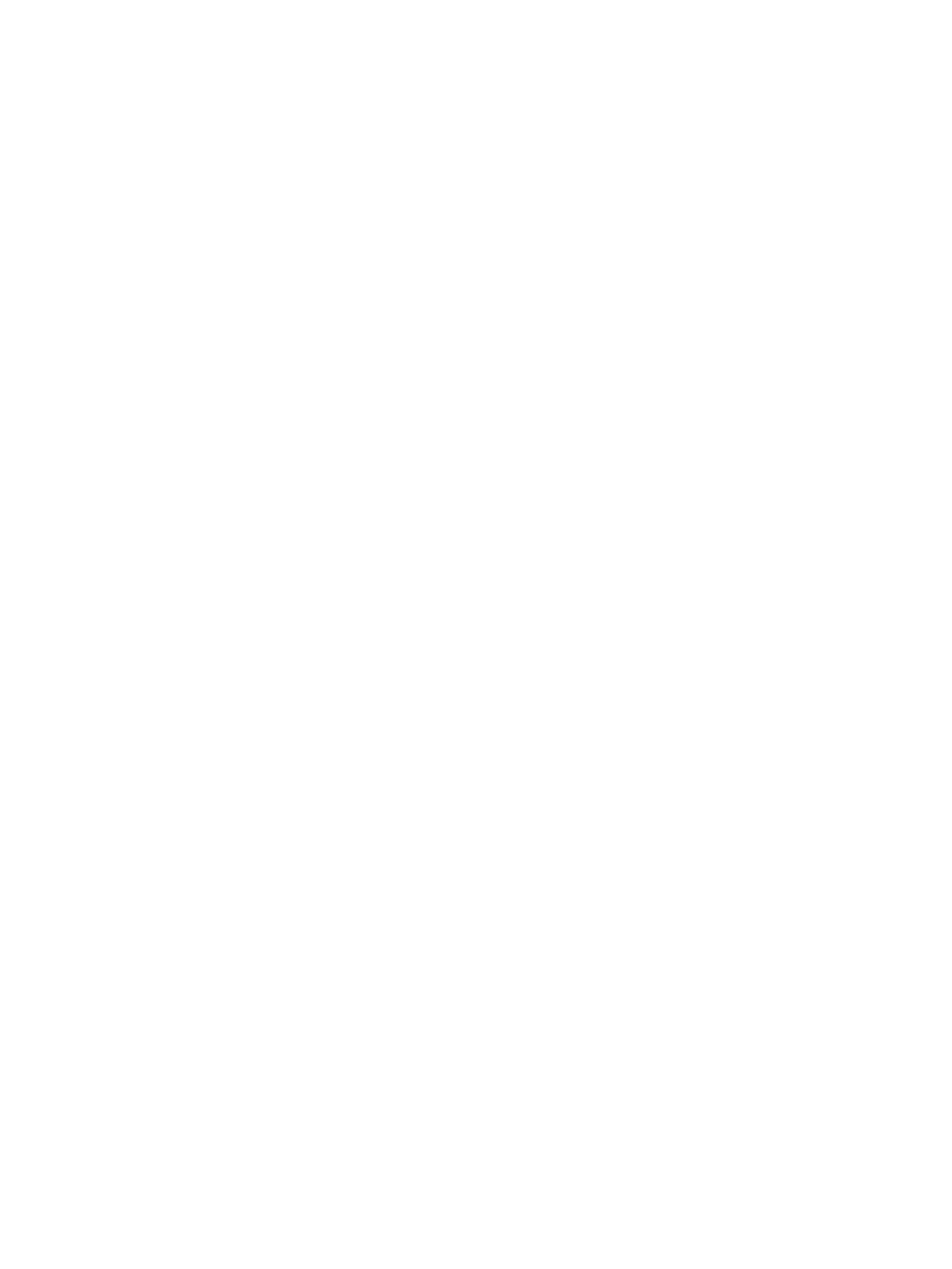
significant than those under normal operations, because
power failure may impose more serious damages to data
centers, such as sudden shutdown of servers and fundamen-
tal destruction of IT equipment. Therefore, emergency cool-
ing is one of the cornerstones to maintain the reliability of
data centers [33–34].
To mitigate these ‘‘mismatches” challenges above, energy stor-
age technologies become inevitable and powerful, which can not
only improve energy utilization efficiency but also balance the dis-
crepancy between supply and demand of energy [35]. Electricity
energy storage and thermal energy storage (TES) are commonly
utilized. Electrical energy storage is an effective way to do
building-grid interaction just as uninterrupted power supply has
been utilized in data center for years, but batteries have drawbacks
like short lifespan, environmental pollution and so on. TES is
another way to achieve the same effect in the long run, being clean
and friendly to the environment.
3. Principles and characteristics of TES
3.1. Principles of TES technologies
In general, TES is divided into physical storage and chemical
storage [36]. Currently, various thermochemical energy storage
materials are at development stage and such a system is not yet
commercially available. What widely used in data centers is phys-
ical energy storage. Physical energy storage is further divided into
sensible thermal energy storage (STES) and latent thermal energy
storage (LTES). The commercial viability of LTES is limited by mate-
rial characteristics and its initial cost, as opposed to STES that is
mostly employed in data center. STES stores thermal energy
through its temperature changes, among which water is commonly
used as the storage material. LTES accumulates thermal energy
almost isothermally, by using phase change material (PCM) such
as ice [37].
STES saves thermal energy by variation of material tempera-
ture, and the storage materials undergo no phase change in the
storage process. The amount of thermal energy stored is propor-
tional to the temperature difference and specific heat capacity dur-
ing charging and discharging processes. It can be defined as,
Q¼Z
T
f
T
i
mC
p
dt ¼mC
ap
T
f
T
i
ð1Þ
where Qis cold energy quantity, kJ/s; T
i
denotes initial temper-
ature, K; T
f
represents final temperature, K; mrefer to flow rate of
fluid, kg/s; C
p
is specific heat capacity, kJ/(kg*K); C
ap
denotes aver-
age specific heat capacity, kJ/(kg*K).
Most materials used in the TES application are inexpensive with
good thermal properties [38]. Concrete, metal, water and air are sen-
sible thermal storage materials usually seen. Water is generally used
as cold energy storage material in data centers, because of its low
price, high specific heat capacity and no pollution or corrosion [39].
LTES stores thermal energy when the storage materials undergo
a phase change process from one physical state to another. The
thermal energy storage capacity includes the portion that varies
with the temperature difference and the portion caused by phase
change of storage materials. The energy formula is given as
follows:
Q¼Z
T
r
T
i
mC
p1
dt þma
r
D
H
r
þZ
T
f
T
r
mC
p2
dt ð2Þ
where Qis cold energy quantity, kJ/s; T
i
denotes initial temper-
ature, K; T
r
represents phase transition temperature, K; T
f
repre-
sents final temperature, K; mrefers to flow rate of fluid, kg/s; C
p1
is specific heat capacity from T
i
to T
r
, kJ/(kg*K); C
p2
is specific heat
capacity from T
r
to T
f
, kJ/(kg*K); a
r
denotes solidified fraction, %;
D
H
r
represents latent heat, kJ/kg.
Phase change materials (PCM) are applied in LTES. PCM are a
group of materials that have an intrinsic capability of absorbing
and releasing heat during phase transition cycles [40]. PCM can
be classified as inorganic PCM including salts, salt hydrates and
alloys, and organic PCM, including paraffin, alcohols, fatty acids,
esters and others. Paraffin, which is mostly applied in data centers,
can be divided into crude paraffin and commercial paraffin. Crude
paraffin has good thermal properties (including transition temper-
ature and latent heat) but its price is high, while commercial paraf-
fin is a good substitute with less inferior thermal parameters, much
lower price and more convenient availability. The exothermic and
endothermic phase transitions of PCM are utilized effectively by
incorporating PCM into TES systems so that thermal loads are
met by controlling the systemic operating parameters. Researchers
are focusing extensively on improving the thermophysical proper-
ties of PCM towards commercialization [41–42]. Heating/cooling
strategies and performance enhancement techniques are practiced
to achieve wide and efficient utilization of PCM in TES systems
[43–44].
Both sensible and latent heat thermal energy storage is utilized
in data center, and could be viewed as substitutes for each other in
some cases. For convenient narration, TES are divided into passive
TES technologies and active TES technologies in this paper. Passive
TES is generally used inside the data center, corresponding to the
cooling terminals and coupled with air flow, while active TES is
integrated with cooling system and generally placed outdoors.
3.2. Characteristic of passive TES
Application of passive TES technologies includes TES embedded
in enclosure [45–47], TES based electronics cooling [48] and ther-
mal mass in data center [49–50]. TES embedded in enclosure and
TES based electronics cooling, often taking PCM as energy storage
materials, are placed dispersedly on the inner surface of enclosure,
and any other locations inside data center considering air flow
arrangement. TES is overcooled by the CRAC system to a low tem-
perature, and release cold energy when the temperature of the
data center increases.
Various thermal masses exist in data center, such as the cold air,
the recirculation air handler coils, supply air ducts, and the raised
metal floor. When the thermal masses in data center are over-
cooled by CRAC system to a lower temperature, they gain the
capacity to absorb heat later as thermal reserve space, to avoid
overheat [49].
From this perspective, thermal masses in data center are viewed
as passive TES, which take indoor air and other substances as sen-
sible energy storage materials. However, due to low specific heat
capacity and limited temperature difference between overcooled
temperature and overheat temperature, the cold energy stored in
these thermal mass is very little.
Energy capacity and heat transfer rate are the most important
parameters we concerned about, thus, materials and configura-
tions of passive TES are overviewed next.
3.2.1. TES materials
Thermal mass in data center requires no special design, and
relies on operation conditions and operation strategies which will
be analyzed in section 6. Different latent heat TES were placed dis-
persedly on the inner surface of enclosure, and any other locations
inside data center to store cold energy. Due to limitation of opera-
tion temperature of the data center, the transition temperature (i.e.
working temperature of TES) were strictly chosen. For passive TES
4L. Liu et al. / Energy & Buildings 226 (2020) 110345