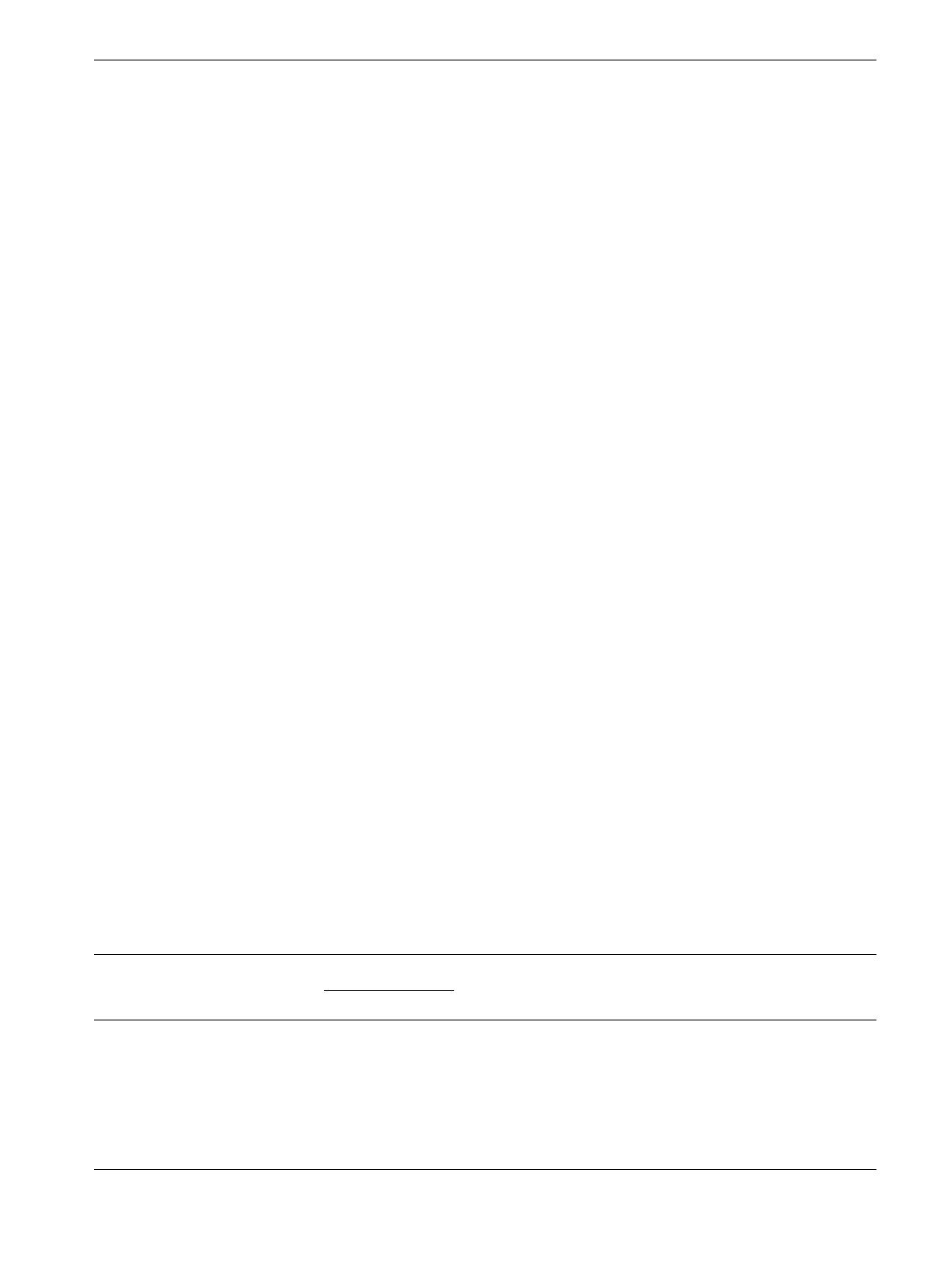
The heterogeneity of milk proteins has contributed
to dif®culties associated with their fractionation and
isolation and, as we acquire more information about
themacromolecules involved, these problems are being
overcome. For more than 50 years, it was thought
that the casein fraction prepared by Hammarsten in
1883 was a pure protein. The application of moving-
boundary electrophoresis by Mellander in 1939 dem-
onstrated the heterogeneity of the casein fraction
with three electrophoretic components denoted as a-,
b-, and g-. Present-day knowledge con®rms that each
of the three components represents more than one
protein. The primary structure and gene sequence
of the four caseins (a
S1
-, a
S2
-, b-, k-) and three of
the whey proteins (b-lactoglobulin, a-lactalbumin,
bovine serum albumin) are now established. The
fractionation and isolation of protein components
depend on the intrinsic physicochemical properties of
the individual proteins. Because some of the proteins
tend to self-associate or associate with other proteins,
a denaturing reaction is required prior to the frac-
tionation stage. Techniques of ultracentrifugation,
size-exclusion chromatography, ultra®ltration and
sodium dodecyl sulphate±polyacrylamide gel elec-
trophoresis (SDS±PAGE) could be adapted for the
separation of certain proteins on the basis of mo-
lecular mass differences. Variability in net electrical
charge, sensitivity to ions, e.g. calcium, and solubility
in the presence of denaturing agents, e.g. urea, could
be exploited for the isolation of some proteins by
precipitation with different concentrations of salt
(ammonium sulphate, calcium chloride), solutions of
alcohol under different pH and temperature condi-
tions. Based on the charge and ionic strength prop-
erties of the proteins, several chromatographic and
electrophoretic procedures have been developed for
their fractionation and isolation. The most appro-
priate procedures for the isolation of milk proteins
depends on the level of purity and the amount (ana-
lytical, preparative, industrial) of protein required.
For a better appreciation of this article, some
familiarity with the nomenclature used for the milk
protein system, as proposed by a committee of the
American Dairy Science Association, is recommended.
Classification and Nomenclature of
Milk Proteins
When raw skim milk is adjusted to pH 4.6, a pre-
cipitate containing approximately 80% of the protein
of cows' milk is formed. This precipitation procedure
has been used as the basis for the classi®cation of
milk proteins into two main groups, with casein in
the precipitate and whey protein (noncasein protein)
inthesupernatant.Table1givesasummaryofsome
of the characteristics of the four types of casein and
the four major proteins in the whey protein fraction.
The primary structure (amino acid sequence) and the
gene sequences of the four caseins, ranging in mol-
ecular mass from 19 038 for k-casein to 25 388 for
a
S2
-casein, have been established. Among the major
whey proteins, a-lactalbumin is the smallest (mol-
ecular mass 14 175 Da) and the immunoglobulins are
the largest (143 000±1 030 000 Da). The complete
amino acid sequences of b-lactoglobulin, a-lac-
talbumin and serum albumin, with molecular masses
of 18 277, 14 175 and 66 267 Da, respectively, are
also known. Unlike b-lactoglobulin and a-lactal-
bumin, serum albumin and some of the immuno-
globulins are not synthesized in the mammary gland.
The immunoglobulins are extremely heterogeneous
and their identities are based on immunochemical
properties. Five classes of immunoglobulins (IgG,
IgA, IgM, IgE, IgD) have been identi®ed in bovine
milk. Their basic structure is similar to other im-
munoglobulins in that they have two heavy and two
light polypeptide chains covalently linked by disul-
phide bonds. The molecular mass varies from 50 to
70 kDa for the heavy chains, depending on the type,
and is about 25 kDa for the light chains. In addition to
Table 1 Characteristics of the major proteins in cows' milk
Protein Molecular mass
a
No. of AA residues No. of
PO
4
Presence of
CH
2
O
Concentration
(g l
ÿ1
)
Genetic variants
detected
Total Pro Cys
a
S1
-Casein 23 164 199 17 0 8 0 10 A, B, C, D, E, F, G, H
a
S2
-Casein 25 388 207 10 2 10±13 0 2.6 A, B, C, D
b-Casein 23 983 209 35 0 5 0 9.3 A
1
,A
2
, A
3
, B, C, D, E, F, G
k-Casein 19 038 169 20 2 1 3.3 A, B, C, E, F
s
, F
I
, G
S
, G
E
, H, I, J
b-Lactoglobulin 18 277 162 8 5 0 0 3.2 A, B, C, D, E, F, H, I, J
a-Lactalbumin 14 175 123 2 8 0 0 1.2 A, B, C
Serum albumin 66 267 582 28 35 0 0 0.4 Ð
Immunoglobulin 1 430 000±1 030 000 8.4% 2.3% Ð 0.8 Ð
a
Molecular mass is for genetic variants in bold.
1882 MILK PROTEINS/Heterogeneity, Fractionation and Isolation
www.alkottob.com
www.alkottob.com