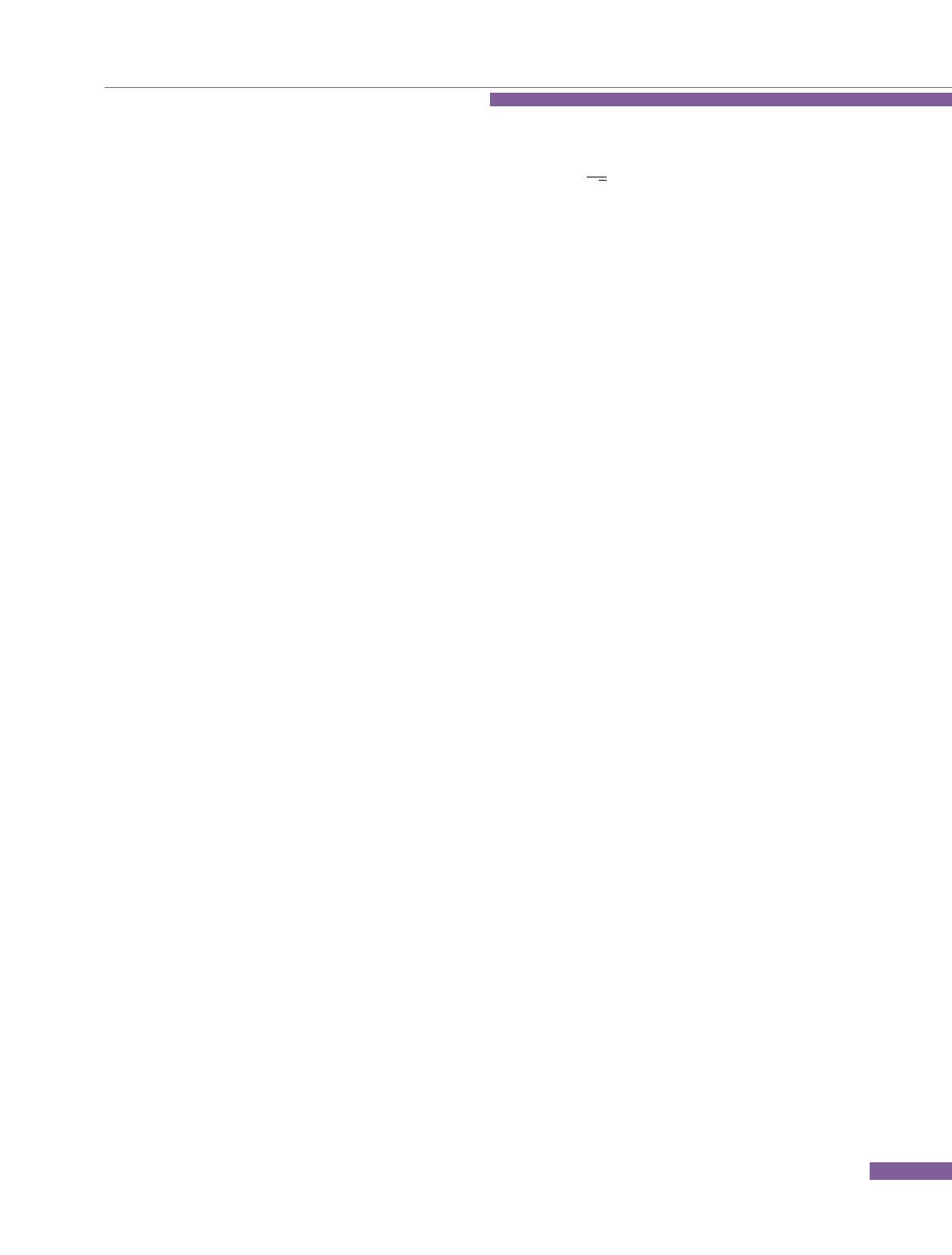
Mariana Duca, Magda Fifirig
1eV[11–16],situationinwhichDRisthedominantprocess
anddissociativeexcitationvanishes. WementiontheDR
measurements for vibrationally cold hydrogen molecular
ions performed in the ion storage ring experiments [17–
26].
Here we continue our previous investigations regarding
theDEofHD+initiallyinthevibrationallevel+
ofthe
electronicgroundstate, withelectrons ofenergy ranging
from2eVto12eV[6]andtheDRandDEindirectmech-
anism,describedbyEq.(2),forH+
2,HD+andDT+[27].
Inthispaper dedicated to the DEprocess for HD+,D+
2,
andDT+we haveappliedour MQDTprocedure[6]con-
sisting of the inclusion of the vibrational continua of the
two lowest electronic states of hydrogen molecular ion
as explicit ionization channels. Sec. 2is devoted to the
presentation of our theoretical approach amenable to a
proper description of the reactive collisions between di-
atomic molecular ions and electrons of energy above the
dissociation threshold of the molecular ion. In order to
simplifyourcomputationshere,wehaveneglectedthero-
tationaleffects,takingintoaccountonlyvibrationalstruc-
ture and interactions. In Sec. 3we extend our model to
HD+,D+
2, and DT+DE cross sections evaluating them
for+
=0and+
=1. Moreexcited states builton the
2σioniccoreareincludedinthiswork. Ananalysisof
thetwoDEmechanismsisalsocarriedout.
2. Basic equations
In the framework of the MQDT [28,29] adapted to DR
processes[30–32] we have performed calculations for the
DEcrosssectionstakingintoaccountthecontributionof
the first excited core of the molecular cation involved in
the electron reactive collision. Here we have achieved a
nonrotationaltreatmentwithcurvecrossing(thepotential
energycurveofadoublyexcitedstatecrossesthepoten-
tialenergycurveoftheionelectronicgroundstateinthe
Franck-Condonregion).
Present theoretical approach consists of the inclusion of
thevibrationcontinuaofthetwolowestelectronicstates
of the molecular cation as explicit ionization channels.
This inclusion yields a good description of the electron-
molecularcationreactivecollisionabovethedissociation
thresholdof the molecular cationground state. Theion-
izationchannels associatedwith thevibrationalcontinua
oftheelectronicstatesofthemolecularcationcorrespond
tostatesobtainedbythediscretizationofthesecontinua.
Eachoftheseionizationchannelsiscorrelatedtoawave
packetχ(R)constructedoftheexactwavefunctionsofthe
continuumspectrum
χ(R)= 1
√δZE+δ/2
E−δ/2χ(RE)E ≥1(4)
E =E0< E1< < E< < EN(5)
wheretheindexdenotestheionizationchannelsassoci-
atedwithvibrationalcontinuaandthepositivequantityδ
is smaller than the energy gap ∆between two consecu-
tivediscretizedlevels(∆=E1−E0= =EN−EN−1),
E beingthedissociationlimitofthecationgroundstate
andENthe superior limit of the energygrid used in the
vibrationalcontinuumdiscretization. Theexactwavefunc-
tions χ(RE)are energy normalized, while the functions
definedby(4)areorthonormalized. Thewavepacket (4)
is practically the eigendifferential [33] of the radial part
χ(RE)ofthecontinuumfunction.
A similar continuum discretization scheme was used by
TakagiintheDEstudyofhydrogenmolecularion[19,20]
andintheDRcomputationofHeH+below1eV[34]. Tak-
agi treated the channels associated with the discretized
levels as dissociation ones. The wave-packet continuum
discretizationmethodwasalsoused forsolvingthescat-
teringofacompositeparticleonatargetnucleus[35,36].
Inthisapproach,twodifferentelectroniccouplingsareac-
complished. Theyareconnectedwiththeinteractionsbe-
tweenthechannelscharacterizingtheelectron-molecular
cation reactive collision, the ionization channels and the
dissociation channels. The ionization channels built on
thegroundioniccore,notedhereby1,arelabeledbythe
pair (), with the vibrational quantum number of the
ground electronic state and the angular quantum num-
beroftheincoming(oroutgoing)electron,whilethedis-
sociationchannelsarelabeledby,thequantumnumber
associatedwith theelectronicdissociativestate. At slow
energiesofthecollidingelectron,thedominantinterchan-
nelinteractionsaretheelectroniccouplingsbetweenthe
ionizationchannelsandthedissociationchannels. These
interactions permit the electron capture into dissociative
channelswithcompetingautoionizationbacktoopenion-
ization channels. The electronic coupling between the
dissociationchannelandtheionizationchannel()is
givenby
V1(R)=hAΦ+
1(+R)φ
(R)|H()|Φ(R)i(6)
whereH()istheelectronicHamiltonian,Atheantisym-
metrizationoperator,theenergyoftheexternalelectron,
and Rthe internuclear distance. The integration is per-
formedoverelectroniccoordinates,whicharedenotedcol-
lectivelybyfortheneutralmoleculeandby+forthe
molecular ion. Φ+
1and Φare the electronic wavefunc-
tionsofthemolecularionstate associatedwiththe ionic
89
Unauthenticated
Download Date | 9/24/15 11:15 PM