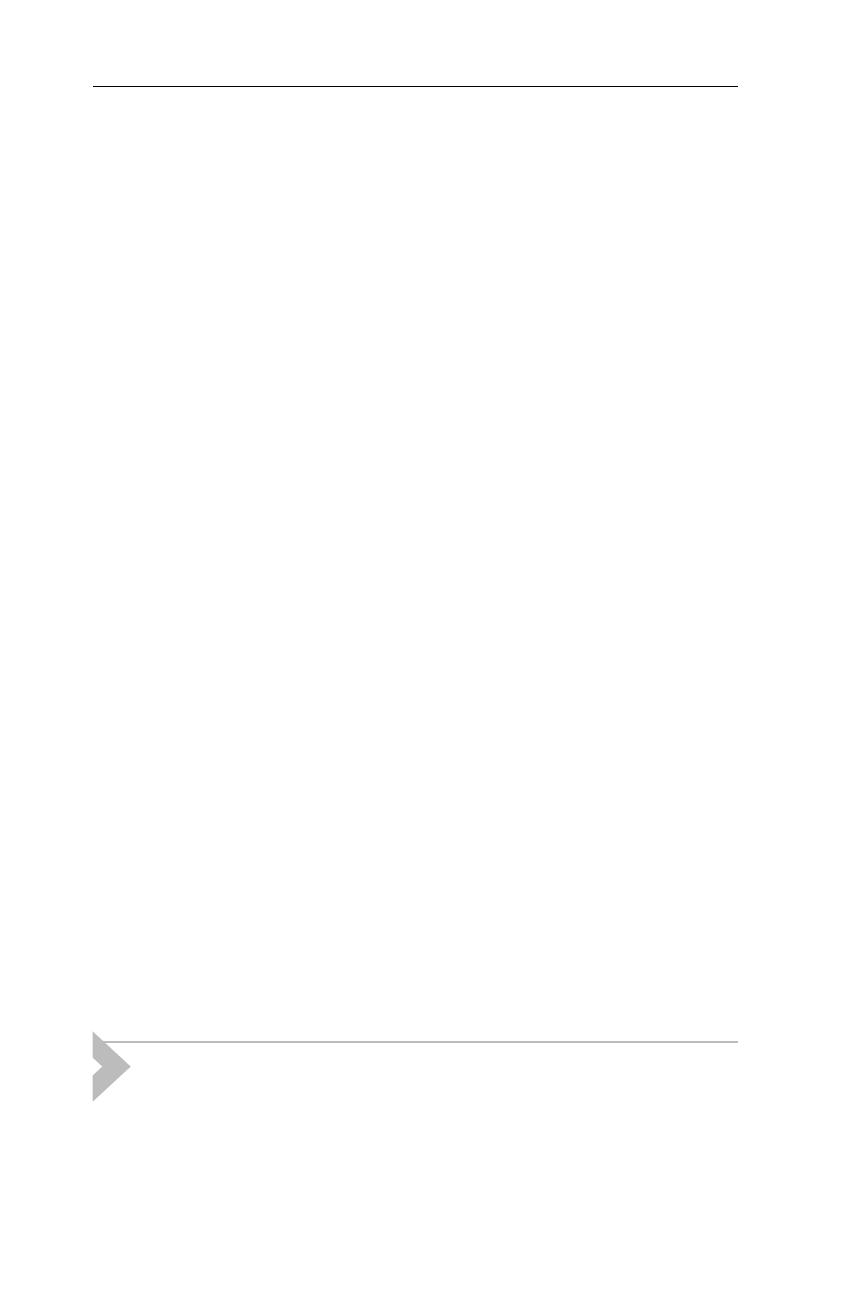
For the X
2
YZ Heusler compounds the situation is completely different
because the two X atoms occupy tetrahedral lattice sites allowing for
magnetic interaction between the X atoms. Thus a second, more deloca-
lized magnetic sublattice is formed in addition to the one originating from
the Y atoms (compare Fig. 1.1). Due to the two different magnetic sub-
lattices, the X
2
YZ Heusler compounds can show all kinds of magnetic
phenomena, and in fact, today ferromagnetism, ferrimagnetism, antiferro-
magnetism, and half-metallic ferromagnetism are known.
Tetragonally distortions corresponding to a type of band Jahn–Teller effect
have been reported in the 1970s (Suits, 1976). Recently, these tetragonally
distorted Heusler compounds have attracted considerable attention (Balke
et al., 2007a; Winterlik et al., 2008; Wu et al., 2009, 2010). The tetragonal
distortion is observed for Mn
2
YZ compounds crystallizing in the inverse
Heusler structure. In this structure, the Mn atoms occupy two different lattice
sites, one with tetragonal and one with octahedral coordination. Theoretical
investigations by Ku
¨bler showed that the Mn atom on the octahedral site
formally possesses an oxidation state of þ3(Mn
3þ
,d
4
)(Ku
¨bler et al., 1983).
According to crystal field theory d
4
high spin ions in an octahedral coordina-
tion sphere undergo a tetragonal distortion, which leads to a rearrangement of
the molecular orbitals and an overall energy gain. This phenomenon is
commonly known as the Jahn–Teller effect. Alternatively, a double degener-
ate van Hove singularity, that is, saddle point in the band structure, can lead to
a tetragonal distortion since this singularity maximizes the band energy,
leading to an unfavorable condition, which is avoided by a tetragonal lattice
distortion. In the case of Mn
2
YZ compounds, the cubic unit cell can undergo
an elongation or a compression along the c-axis. Consequently, the symmetry
of the crystal changes from the cubic space group F
43mto the tetragonal space
group I
4m2. Up two now, only few tetragonally distorted Heusler materials
have been studied thoroughly, Mn
3x
Ga being the most prominent example
(Balke et al., 2007a; Winterlik et al., 2008). These materials are particularly
interesting due to the perpendicular magnetic anisotropy (PMA), which can
be achieved in thin films (Wu et al., 2009) opening the door to spin-transfer
torque (STT) devices. Many potential compounds Mn
2
YZ and their alloys
Mn
3x
Y
x
Z for a STT application are still undiscovered. However, these
materials still exhibit certain challenges, which have to be overcome for
technological application. It is therefore essential to design new materials
that fulfill all the required criteria as we will discuss later.
2. Crystal Structure and Atomic Ordering
The Half-Heusler compounds have the general formula XYZ and
crystallize in a noncentrosymmetric cubic structure (space group no. 216,
F
43m,C1
b
) which is a ternary ordered variant of the CaF
2
structure and can
4Tanja Graf et al.