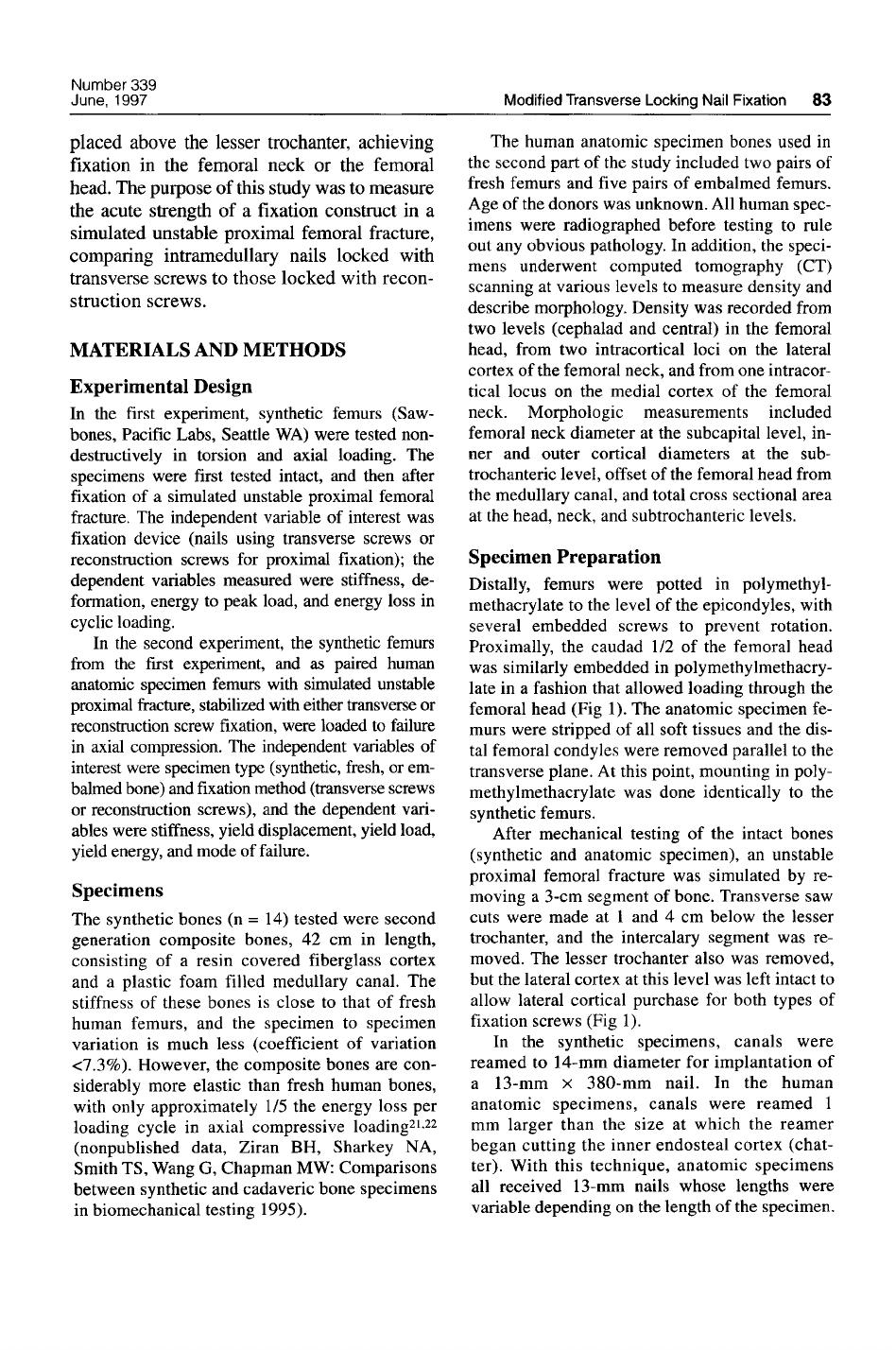
Number 339
June, 1997 Modified Transverse Locking Nail Fixation
83
placed
above
the
lesser
trochanter, achieving
fixation
in
the
femoral
neck
or
the
femoral
head.
The
purpose
of this
study
was
to
measure
the
acute
strength
of
a
fixation
construct
in
a
simulated unstable
proximal
femoral fracture,
comparing intramedullary
nails
locked
with
transverse
screws
to
those locked with recon-
struction screws.
MATERIALS AND METHODS
Experimental Design
In the first experiment, synthetic femurs (Saw-
bones, Pacific Labs, Seattle
WA)
were tested non-
destructively in torsion and axial loading. The
specimens were first tested intact, and then after
fixation of a simulated unstable proximal femoral
fracture. The independent variable of interest was
fixation device (nails using transverse screws
or
reconstruction screws for proximal fixation); the
dependent variables measured were stiffness, de-
formation, energy to peak load, and energy
loss
in
cyclic loading.
In the second experiment, the synthetic femurs
from the first experiment, and
as
paired human
anatomic specimen femurs with simulated unstable
proximal fracture, stabilized with either transverse or
reconstruction screw fixation, were loaded to failure
in axial compression. The independent variables of
interest were specimen type (synthetic, fresh, or em-
balmed bone) and fixation method (transverse screws
or reconstruction screws), and the dependent vari-
ables were stiffness, yield displacement, yield load,
yield energy, and mode of failure.
Specimens
The synthetic bones (n
=
14) tested were second
generation composite bones,
42
cm in length,
consisting of a resin covered fiberglass cortex
and a plastic foam filled medullary canal. The
stiffness of these bones is close to that of fresh
human femurs, and the specimen to specimen
variation is much less (coefficient of variation
<7.3%). However, the composite bones are con-
siderably more elastic than fresh human bones,
with only approximately
1/5
the energy
loss
per
loading cycle in axial compressive loading2L.22
(nonpublished data, Ziran
BH,
Sharkey
NA,
Smith TS, Wang
G,
Chapman
MW
Comparisons
between synthetic and cadaveric bone specimens
in biomechanical testing 1995).
The human anatomic specimen bones used
in
the second part of the study included two pairs
of
fresh femurs and five pairs of embalmed femurs.
Age of the donors was unknown. All human spec-
imens were radiographed before testing to rule
out any obvious pathology. In addition, the speci-
mens underwent computed tomography
(CT)
scanning at various levels to measure density and
describe morphology. Density was recorded from
two levels (cephalad and central) in the femoral
head, from two intracortical loci
on
the lateral
cortex of the femoral neck, and from one intracor-
tical locus on the medial cortex of the femoral
neck. Morphologic measurements included
femoral neck diameter at the subcapital level, in-
ner and outer cortical diameters at the sub-
trochanteric level, offset
of
the femoral head from
the medullary canal, and total cross sectional area
at the head, neck, and subtrochanteric levels.
Specimen Preparation
Distally, femurs were potted in polymethyl-
methacrylate to the level of the epicondyles, with
several embedded screws to prevent rotation.
Proximally, the caudad
1/2
of the femoral head
was similarly embedded in polymethylmethacry-
late in a fashion that allowed loading through the
femoral head (Fig
1).
The anatomic specimen fe-
murs were stripped of all soft tissues and the dis-
tal femoral condyles were removed parallel to the
transverse plane. At this point, mounting
in
poly-
methylmethacrylate was done identically to the
synthetic femurs.
After mechanical testing of the intact bones
(synthetic and anatomic specimen), an unstable
proximal femoral fracture was simulated by re-
moving a 3-cm segment of bone. Transverse saw
cuts were made at 1 and
4
cm below the lesser
trochanter, and the intercalary segment was re-
moved. The lesser trochanter also was removed,
but the lateral cortex at this level was left intact to
allow lateral cortical purchase for both types of
fixation screws (Fig
1).
In the synthetic specimens, canals were
reamed to 14-mm diameter for implantation of
a 13-mm
x
380-mm nail. In the human
anatomic specimens, canals were reamed
1
mm larger than the size at which the reamer
began cutting the inner endosteal cortex (chat-
ter). With this technique, anatomic specimens
all received 13-mm nails whose lengths were
variable depending on the length of the specimen.