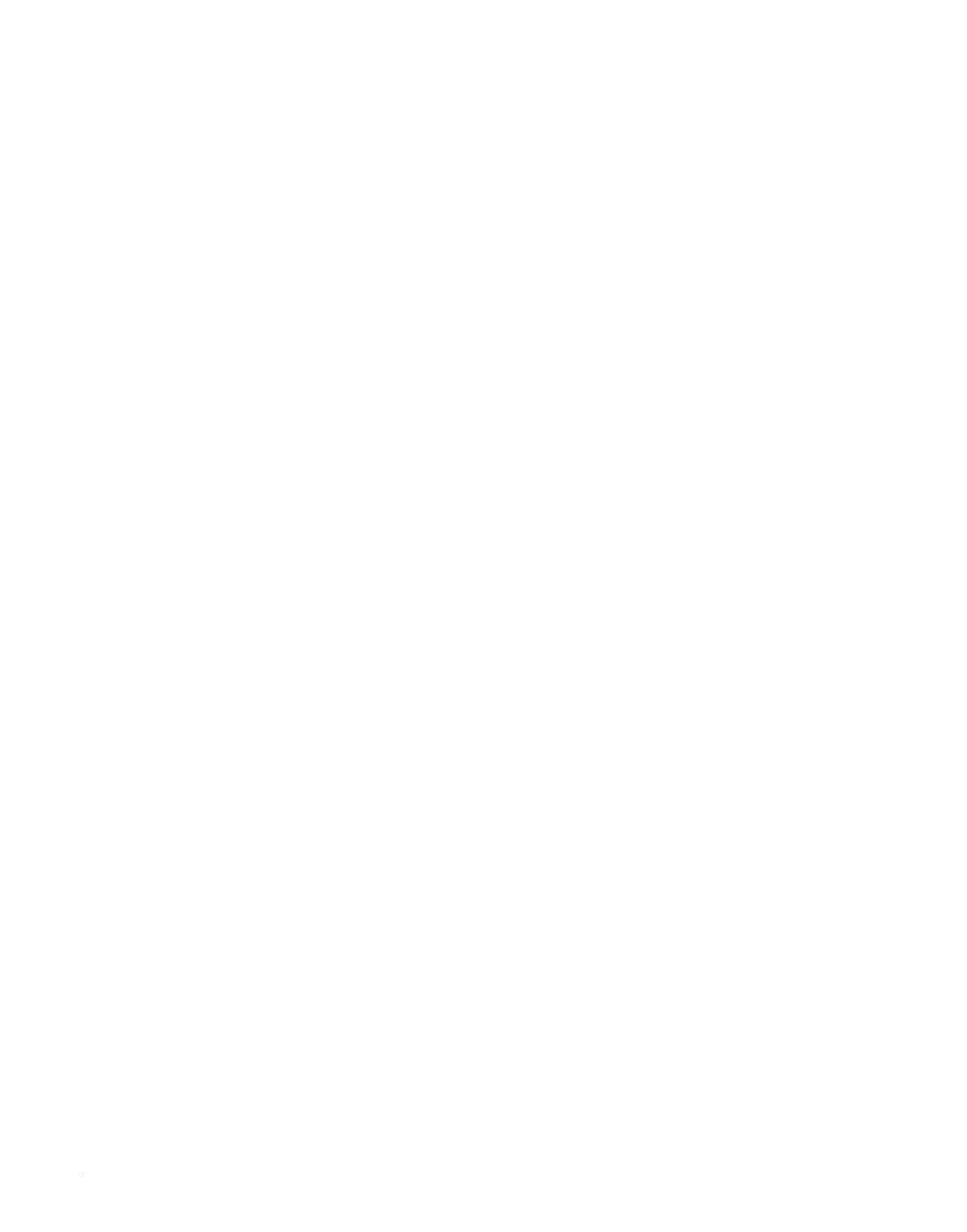
()
M.V.L. BennettrBrain Research ReÕiews 32 2000 16–28 17
40 years ago, is pretty well understood, not completely of
course, but there are no very large black boxes. It seems
much more like yesterday, and is, that I last wrote a review
on the development of knowledge of electrical transmis-
wx
sion 73 . What has happened in the last couple of years?
Aside from substantial but incremental progress, there has
wx
been the discovery of neuron specific connexins 26,63
and of connexin diseases that involve the nervous system
wx
15,22,38 . Although no diseases of electrical synapses
have been discovered yet, there are sure to be mutations of
neuron specific connexins, whether or not the mutations
lead to an observable phenotype. Interestingly, mutations
of Cx26 and Cx32, which are expressed by some neurons
in the CNS, have yet to show any phenotypic changes in
those neurons. Gene targeting is progressing through the
connexin gene family. And there is the discovery that gap
Ž.
junctions of the Ecdysozoa the nematode, arthropod line
are encoded by a gene family completely unrelated to the
Ž
connexins, although there are many convergent not con-
.
served properties between the junctions encoded by that
gene family and by the connexins. Since the new connex-
ins and connexin diseases are extensively discussed in this
volume by others more directly involved in the research,
and I just participated in writing a review of connexin
wx
diseases 15 , I will make this presentation more an oral
history than an integrative summary and analysis.
One does not have to have been at Oxford to define a
synapse as a specialized site of functional interaction
Ž.
between neurons, although Sherrington and Eccles and I
were. By this definition gap junctions form one class of
wx
electrical synapse 13 . Another kind of electrical synapse
mediates short latency inhibition of the Mauthner cell of
teleost fishes and possibly mammalian cerebellar Purkinje
cells; this form of electrical transmission is not mediated
by gap junctions, and involves different junctional special-
Ž.wx
izations see below 28 . In addition, there probably are
electrical interactions that occur between closely apposed
cells without obvious gap junctions or specializations other
wx
than the absence of interposed glia 28,36,67 . Whether
these sites are to be considered synapses, i.e., specialized,
or ephapses, i.e., incidental or accidental sites of interac-
tion, may become clear with greater knowledge of the
developmental mechanisms. Without deciding on a name
one can still describe the electrical interaction, which does
indeed appear to be uniquely associated with the close
appositions.
2. An aside on ‘‘ephapses’’ and other nomenclatural
niceties
wx
Angelique Arvanitaki 1 coined the term ephapse from
the Greek to mean an apposition that is not quite so close
Ž
as a synapse according to my Greek–American colleague,
.
George Dimitrios Pappas . She used it to denote what she
thought of as artificial synapses, which she made by laying
one axon along side another where the ‘‘action currents’’
generated during an impulse in one axon altered the ex-
citability of the other axon. This terminology suggests that
she thought synaptic transmission was electrical, at least
that is my recollection, and, in the spirit of oral history, I
will not go to the library to try to confirm that view.
Ephapse then came to be used for incidental contacts in the
nervous system, particularly where activity in one or more
axons excited other axons. These days one might wonder if
actual gap junction electrical synapses were formed be-
tween axons in injured tissue.
My mentor at that time, Harry Grundfest, had embraced
the idea that chemical transmission was mediated at elec-
trically inexcitable membrane, i.e., in explicit, modern
terms that the conductance of the neurotransmitter recep-
tors was independent of membrane potential and only a
function of transmitter concentration. To keep transmission
at synapses chemically pure, he decided to use ephapse to
denote morphological specializations between neurons
where transmission was electrical. Although my Oxford
education led me to disagree with this practice, to keep
peace in the laboratory I used ‘‘electrotonic junctions’’ for
one type of what I now freely term electrical synapses. At
that time I felt that ephapse connoted artificiality or inci-
dentality, which downgraded the importance of my work.
Electron microscopy now makes it clear that gap junctions
are closer appositions than occur at chemical synapses,
and, if one were starting over, one would call chemical
synapses ephapses and gap junctions between neurons
Ž.
synapses. In my view both he and Jack later Sir John
Eccles made a mistake in thinking that only one mode of
transmission could be synaptic, but Eccles was better at
wx
changing his mind 14 .
I recall when David Potter presented his and Edward
wx
Furshpan’s work 31 on the crayfish giant motor synapse
at the Monday night electrobiology seminars at the Marine
Ž.
Biological Laboratory MBL, Woods Hole . This work and
wx
the independent studies of Akira Watanabe 70 on the
cardiac ganglion of the mantid shrimp were the first
unequivocal demonstrations of electrical transmission be-
tween neurons. The electrobiology sessions were organized
by Harry, who welcomed airing of all views, and were
known as the Monday Night Fights because of the some-
times heated discussion and by analogy with the Friday
Night Fights, a popular program at the time showing
professional boxing matches. Harry suggested in the dis-
cussion period, or possibly before, since interruptions were
not uncommon, that they should call their rectifying
synapse an ephapse, because it was electrical although it
was electrically inexcitable. David pointed out to him with
evident pleasure that the junctional conductance was a
function of voltage and thus was electrically excitable.
Harry did not have a good retort, which was unusual for
him. Of course gap junctions between segments of the
septate axon are electrically linear over a wide range
wx
37,71 , while connexin based gap junctions all show some