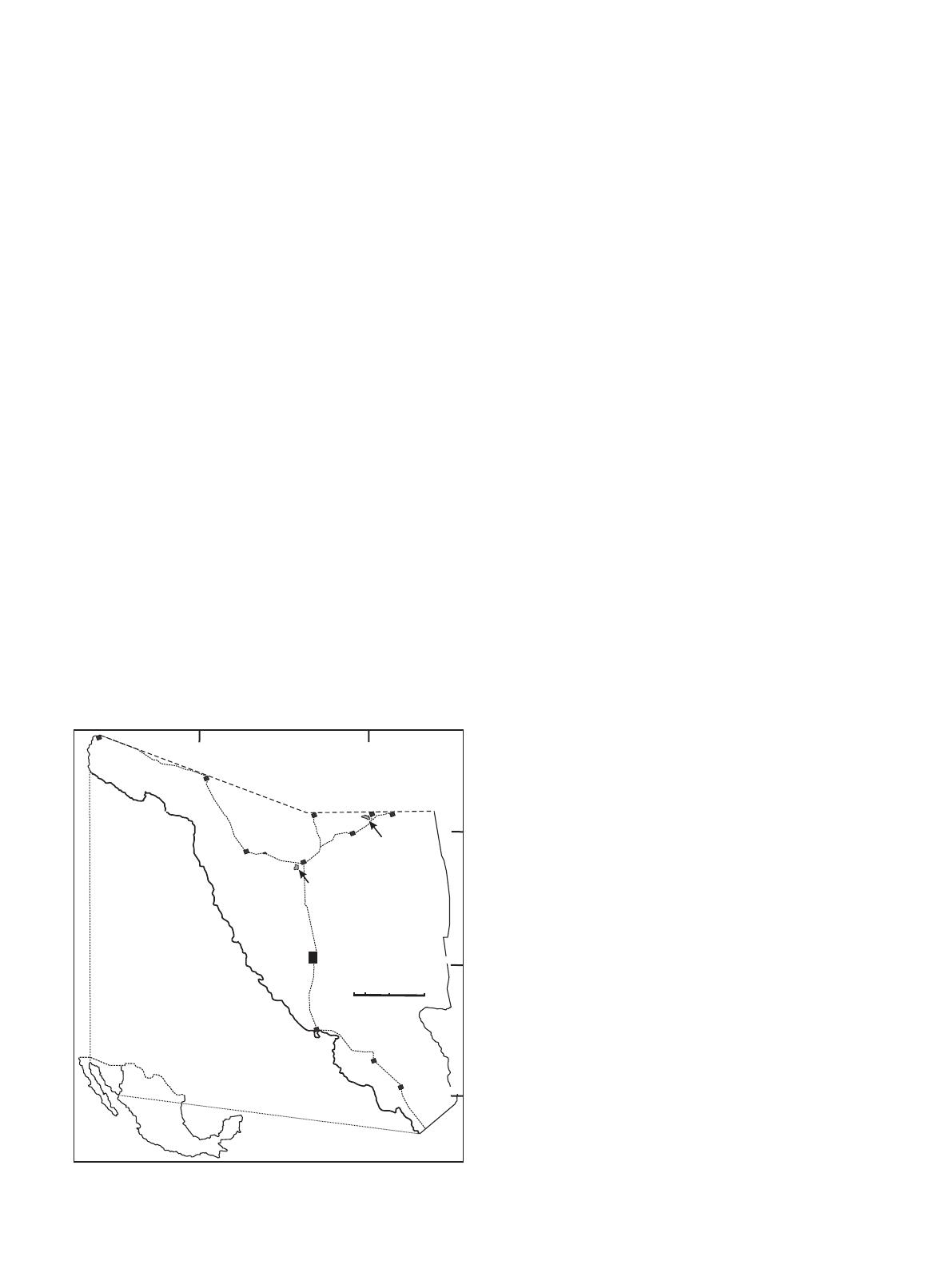
The distribution of REEs and Ce anomalies in marine sediments
may be influenced by depositional environments such as proximity
to source area (Murray et al., 1991a), widespread marine anoxia (Liu
et al., 1988; German and Elderfield, 1990; Murray et al., 1991b),
surface productivity variation (Toyoda et al., 1990), oceanic redox
conditions (Liu et al., 1988; German and Elderfield, 1990) and
lithology and diagenesis (Nath et al., 1992; Madhavaraju and
Ramasamy, 1999; Armstrong-Altrin et al., 2003; Madhavaraju and
Lee, 2009). Ce anomalies in marine sediments are considered by
some as reliable indicators for understanding the paleoredox
conditions (Liu et al., 1988), although several workers have raised
doubts about their effectiveness (German and Elderfield, 1990;
Murray et al., 1991b; Nath et al., 1992, 1997).
The REE signatures in ancient marine environment provide
information on secular changes in detrital influx and oxygenation
conditions in the water column (e.g. Holser, 1997; Kamber and
Webb, 2001). The seawater signatures are, however, completely
masked by the incorporation of terrigenous materials, which have
relatively high, non-seawater-like REE contents (Murray et al.,1992;
Webb and Kamber, 2000; Nothdurft et al., 2004; Madhavaraju and
Lee, 2009). The identification of the terrigenous particles present
in the marine carbonate rocks as contaminants is an important
aspect to understand the geochemistry of carbonate rocks.
The Lower Cretaceous, shallow marine siliciclastic and calcar-
eous strata of the Mural Formation are exposed in northern Sonora,
northwest Mexico in a 300 km long transect that extends from
Sierra El Chanate (westernmost part) to Cerro El Caloso Pitaycachi
(northeastern most outcrop). Along this transect, González-León
et al. (2008) reported the stratigraphy and biostratigraphy of
several sections, including the Cerro Pimas and Sierra San José
sections (Figs. 1 and 2) of which we discuss herein the major, trace
and REE geochemistry of their limestone beds. The aims of our
study are to determine the influence of terrigenous materials on the
REE characteristics of carbonate rocks, to document the variations
in Ce anomalies and to unravel the probable reason for significant
positive Eu anomalies in the limestones of the Mural Formation.
2. Geology and Stratigraphy
The Lower Cretaceous sedimentary succession assigned to the
Bisbee Group is well exposed in the north-central part of the state
of Sonora, Mexico. This succession has similar stratigraphic and
lithologic characteristics to the younger formations of the Bisbee
Group (Ransome, 1904) of southern Arizona and New Mexico in the
United States of America, and is correlative with strata exposed in
northern Mexico (Cantu-Chapa, 1976; Bilodeau and Lindberg, 1983;
Mack et al., 1986; Dickinson et al., 1989; Jacques-Ayala, 1995;
Lawton et al., 2004). In Arizona the Bisbee Group consists of the
Glance Conglomerate and the Morita, Mural and Cintura Forma-
tions that were deposited in a rift basin, termed the Bisbee Basin.
The older unit is the Glance Conglomerate composed of cobble- to
boulder-conglomerate with local interbeds of volcanic flows and
tuffs, which represent syntectonic rift deposits (Bilodeau et al.,
1987; Lawton et al., 2004).
The Morita and Cintura Formations are composed of reddish
brown siltstone and lenticular beds of arkose and feldspathic
arenite (Dickinson et al., 1986; Klute, 1991)that were deposited
under fluvial conditions. These two formations are difficult to
distinguish based only on their lithological characteristics. Hence,
the intervening marine Mural Formation is key to understanding
Lower Cretaceous stratigraphy and basin configuration in the area.
The fossiliferous clastic and carbonate strata of the Mural
Formation were deposited during a major marine transgression
during AptianeAlbian time (Scott, 1987) in the region of Sonora and
Arizona where it overlies the Morita Formation on a sharp ravine-
ment surface and grades upward into the Cintura Formation.
Lawton et al. (2004) defined six members in the Mural Formation in
north-central Sonora (Fig. 2), which from the base upwards are the
Cerro La Ceja, Tuape Shale, Los Coyotes, Cerro La Puerta, Cerro La
Espina and Mesa Quemada members. The lithostratigraphic studies
of different members of the Mural Formation show minor facies
changes from west to east. The facies characteristics and regional
correlation of different members of the Mural Formation indicate
that the depositional environments of this formation varied from
restricted shelf with deltaic and fluvial influence to open shelf with
coral rudist buildups, to offshore shelf. For the present study, we
have collected limestone samples from the western part (Cerro
Pimas eCP) and the eastern part (Sierra San José eSSJ) of the
Bisbee Basin in northern Sonora. Here the limestones of the Mural
Formation were deposited in a nearshore environment with deltaic
and fluvial influence to open marine environments (González-León
et al., 2008). Most of the limestone samples contain varied amounts
of terrigenous materials.
The Cerro La Ceja (CLC) Member consists of interbedded bio-
clastic limestone, siltstone and calcareous sandstone. The limestone
beds are grey, brown and dark yellowish brown, bioturbated and
locally sandy. Siltstone beds are grey, green and reddish brown with
calcareous nodules. The Tuape Shale (TS) Member is mainly
composed of grey to black mudstone and shale, shaly limestone and
subordinate amount of siltstone and fine grained sandstone.
Limestone occurs as thin beds which contain oysters and ammo-
nites. The Los Coyotes (LC) Member consists of thin beds of brown
mudstone, calcareous siltstone, shaly limestone, massive brown
siltstone, fine-grained sandstone and bioclastic limestone. This
member contains abundant fossils such as oysters, trigoniids,
gastropods, bivalves and echinoderms. The Cerro La Puerta (CLP)
Member is composed of mostly black shale and thin beds of fine-
grained sandstone and fossiliferous limestone. The limestone
exhibits distinct bedding-parallel burrows on the upper bed
surfaces, and it contains fossils including oysters and Orbitolina; the
black shale contains calcareous nodules. The Cerro La Espina (CLE)
Member consists mainly of massive limestone with thin beds of
SONORA ARIZONA
HERMOSILLO
Santa Ana
Caborca
Sonoita
San Luis
R. C.
Nogales
Cananea
Agua Prieta
Naco
Cerro
Pimas
Sierra
San Jose
Guaymas
Obregon
Navojoa
060 12020
Km
Mexico
113 00 110 00
31 00
29 00
27 00
Fig. 1. Location map of the studied sections of the Mural Formation.
J. Madhavaraju et al. / Cretaceous Research 31 (2010) 400e414 401