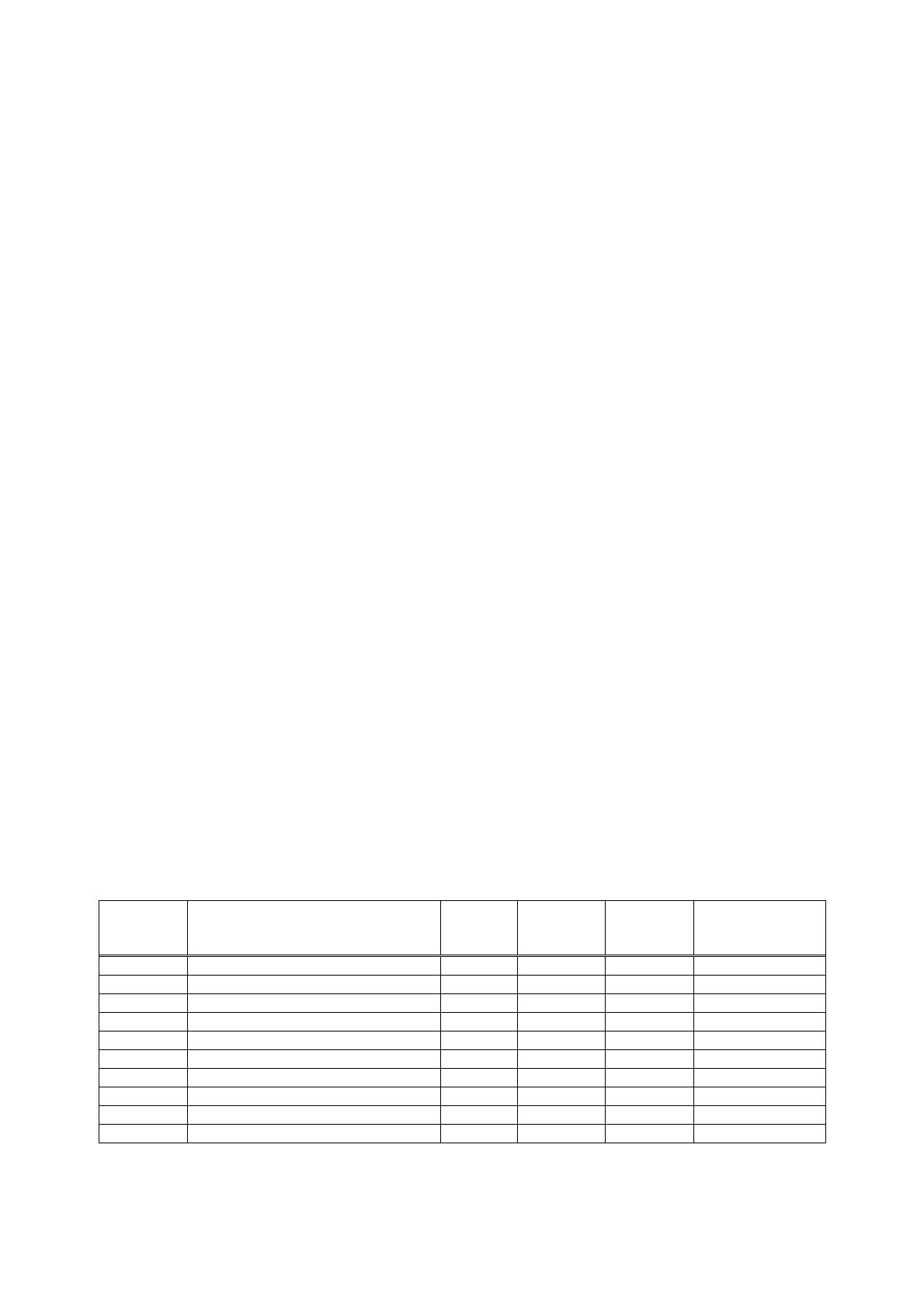
leachates (Lesage et al., 1990; Denovan and Strand, 1992; Lesage et al., 1992) and laboratory
municipal waste digesters (Deipser and Stegmann, 1994; Deipser and Stegmann, 1997;
Deipser, 1998), enzymatic reductive dechlorination of CFC-113 was observed leading to the
formation of HCFC-123a, chlorotrifluoroethene (CTFE), and two isomers of HCFC-133.
The reductive dehalogenation of the HCFCs has received less attention. HCFC-21 and HCFC-
123 were reported to be enzymatically dechlorinated under anaerobic conditions in freshwater
and salt marsh sediments (Oremland et al., 1996). A disruption of fluorine-carbon bonds
under environmental conditions has not been observed so far neither in CFCs nor in HCFCs
(Key et al., 1997). No data on dehalogenation of HFC-134a, HCFC-141b, HCFC-142b which
are currently in use as replacement products for CFCs, are reported. Also, no comparative
study has been reported so far on the dehalogenation of CFCs relative to HCFCs.
Two major processes determine the fate of (H)CFCs in groundwater: volatilization and
biodegradation. A parallel study showed the importance of water table fluctuations on the
volatilization of these compounds from the saturated zone (Werner and Höhener, 2002). This
process can represent a non negligible contribution to the removal of (H)CFC’s from
groundwater.
The aim of the present study was to investigate the potential reductive dehalogenation of the
nine most widely used CFCs and HCFCs in a methanogenic enrichment culture from sewage
sludge. The compounds were chosen from the production data (see Fig.1) published by the
Alternative Fluorocarbons Environmental Acceptability Study (AFEAS), an association of the
leading manufacturers of CFCs and HCFCs.
Materials and methods
Chemicals
(Hydro)chlorofluorocarbons CFC-11, CFC-12, CFC-114, HCFC-21, HCFC-22, HCFC-142b
and HFC-134a were obtained from Fluka (Buchs, Switzerland) in the highest available
purity. CFC-113 was obtained from Merck (Dietikon, Switzerland). HCFC-141b was
obtained from Prochimac SA (Neuchâtel, Switzerland). CFC-115 was obtained from a
refrigeration system (unknown manufacturer). The relevant physical-chemical properties of
the compounds are given in Table 1. All water used was distilled and was 18 megaohm
resistance or greater.
Table 1: Chemical properties, industrial use and experimental parameters of the CFCs and
HCFCs used in this study.
Short Name Use1) Henry GC Initial
name coefficient retention concentration
[mol/l*atm] time [min] in solution [nM]
CFC-11 Trichlorofluoromethane R, I, A 0.01042) 4.18 365
CFC-12 Dichlorodifluoromethane R, I, A 0.002912) 2.19 371
HCFC-21 Dichlorofluoromethane - 0.08443) 4.09 4540
HCFC-22 Chlorodifluoromethane R 0.03194) 2.13 2540
CFC-113 1,1,2-Trichloro-1,2,2-trifluoroethane S 0.0024) 8.40 99
CFC-114 1,2-Dichloro-1,1,2,2-tetrafluoroethane A 0.000834) 3.23 80
CFC-115 Chloropentafluoroethane R 0.000384) 1.93 89
HFC-134a 1,1,1,2-Tetrafluoroethane R 0.025) 2.42 1780
HCFC-141b 1,1-Dichloro-1-fluoroethane I, A, S 0.00796) 7.80 2257
HCFC-142b 1-Chloro-1,1-difluoroethane I 0.0146) 3.40 2500
1) Refrigerant; I: Insulation foam; A: Aerosol; S: Solvent; 2) Warner and Weiss, 1985; 3) VP/WSOL;
4) Yaws et al., 1991; 5) Chang and Criddle, 1995; 6) Kanakidou et al., 1995.
3