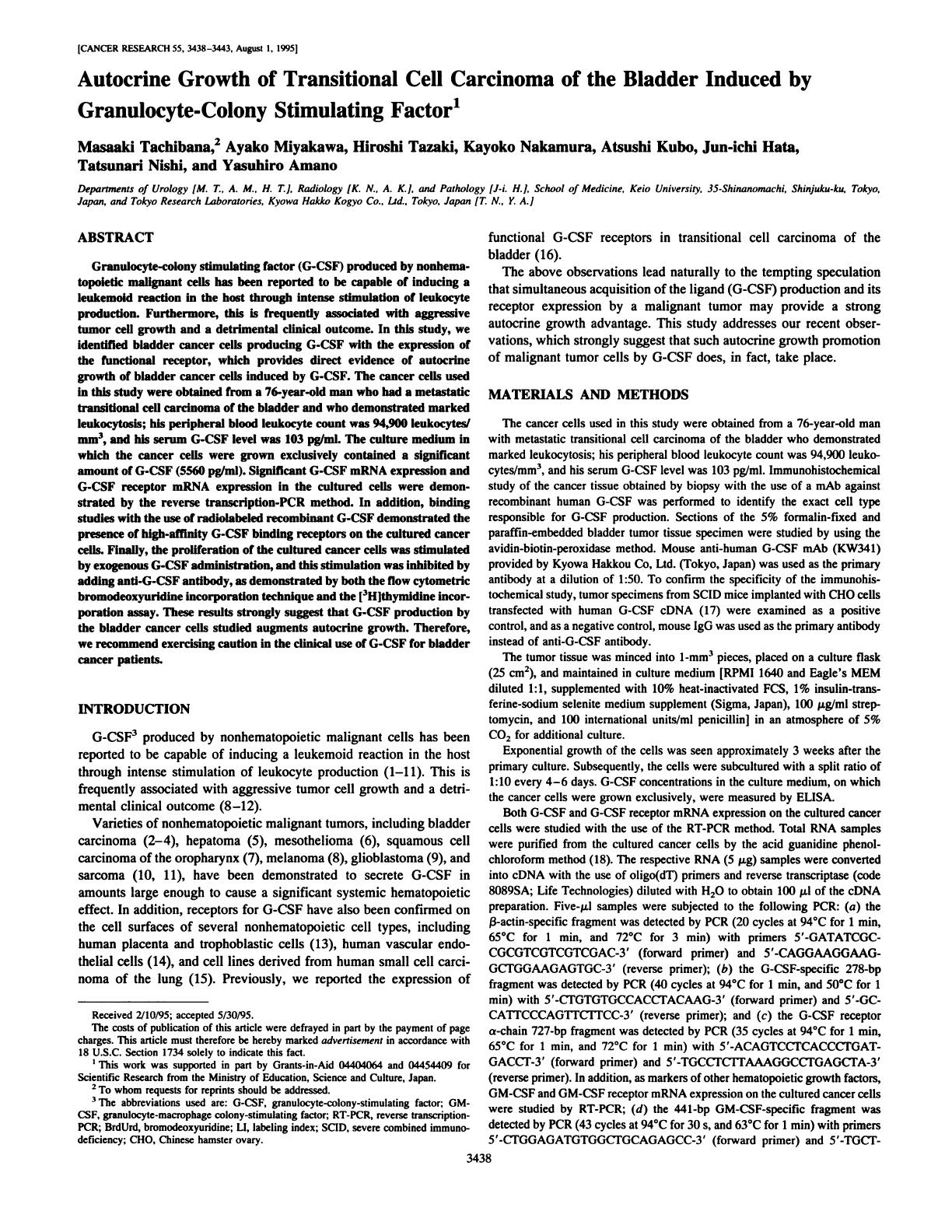
(CANCER RESEARCH 55, 3438-3443. August I. 1995]
Autocrine Growth of Transitional Cell Carcinoma of the Bladder Induced by
Granulocyte-Colony Stimulating Factor1
Masaaki Tachibana,2 Ayako Miyakawa, Hiroshi Tazaki, Kayoko Nakamura, Atsushi Kubo, Jun-ichi Hata,
Tatsunari Nishi, and Yasuhiro Amano
Departments of Urology ¡M.T.. A. M., H. T.], Radiology [K. N., A. K.I, and Pathology fj-i. ti.}. School of Medicine, Keio University, 35-Shinanomachi. Shinjukit-ku, Tokyo,
Japan, and Tokyo Research Laboratories, Kyowa Hakko Kogyo Co., Liti., Tokyo, Japan [T. N., Y. A.]
ABSTRACT
Granulocyte-colony stimulating factor (G-CSF) produced by nonhema-
topoietic malignant cells has been reported to be capable of inducing a
leukemoid reaction in the host through intense stimulation of leukocyte
production. Furthermore, this is frequently associated with aggressive
tumor cell growth and a detrimental clinical outcome. In this study, we
identified bladder cancer cells producing G-CSF with the expression of
the functional receptor, which provides direct evidence of autocrine
growth of bladder cancer cells induced by G-CSF. The cancer cells used
in this study were obtained from a 76-year-old man who had a metastatic
transitional cell carcinoma of the bladder and who demonstrated marked
leukocytosis; his peripheral blood leukocyte count was 94,900 leukocytes/
mm3, and his serum G-CSF level was 103 pg/ml. The culture medium in
which the cancer cells were grown exclusively contained a significant
amount of G-CSF (5560 pg/ml). Significant G-CSF inKN A expression and
G-CSF receptor mRNA expression in the cultured cells were demon
strated by the reverse transcription-PCR method. In addition, binding
studies with the use of radiolabeled recombinant G-CSF demonstrated the
presence of high-affinity G-CSF binding receptors on the cultured cancer
cells. Finally, the proliferation of the cultured cancer cells was stimulated
by exogenous G-CSF administration, and this stimulation was inhibited by
adding anti-G-CSF antibody, as demonstrated by both the flow cytometric
bromodeoxyuridine incorporation technique and the | 'lI |lh\miilinc incor
poration assay. These results strongly suggest that G-CSF production by
the bladder cancer cells studied augments autocrine growth. Therefore,
we recommend exercising caution in the clinical use of G-CSF for bladder
cancer patients.
INTRODUCTION
G-CSF3 produced by nonhematopoietic malignant cells has been
reported to be capable of inducing a leukemoid reaction in the host
through intense stimulation of leukocyte production (1-11). This is
frequently associated with aggressive tumor cell growth and a detri
mental clinical outcome (8-12).
Varieties of nonhematopoietic malignant tumors, including bladder
carcinoma (2-4), hepatoma (5), mesothelioma (6), squamous cell
carcinoma of the oropharynx (7), melanoma (8), glioblastoma (9), and
sarcoma (10, 11), have been demonstrated to secrete G-CSF in
amounts large enough to cause a significant systemic hematopoietic
effect. In addition, receptors for G-CSF have also been confirmed on
the cell surfaces of several nonhematopoietic cell types, including
human placenta and trophoblastic cells (13), human vascular endo-
thelial cells (14), and cell lines derived from human small cell carci
noma of the lung (15). Previously, we reported the expression of
Received 2/10/95; accepted 5/30/95.
The costs of publication of this article were defrayed in part by the payment of page
charges. This article must therefore he hereby marked advertisement in accordance with
18 U.S.C. Section 1734 solely to indicate this fact.
1This work was supported in part by Grants-in-Aid 04404064 and 04454409 for
Scientific Research from the Ministry of Education, Science and Culture. Japan.
2 To whom requests for reprints should be addressed.
'The abbreviations used are: G-CSF, granulocyte-colony-stimulating factor; GM-
CSF. granulocyte-macrophage colony-stimulating factor; RT-PCR, reverse transcription-
PCR; BrdUrd, bromodeoxyuridine; LI, labeling index; SCID, severe combined immuno
deficiency; CHO, Chinese hamster ovary.
functional G-CSF receptors in transitional cell carcinoma of the
bladder (16).
The above observations lead naturally to the tempting speculation
that simultaneous acquisition of the ligand (G-CSF) production and its
receptor expression by a malignant tumor may provide a strong
autocrine growth advantage. This study addresses our recent obser
vations, which strongly suggest that such autocrine growth promotion
of malignant tumor cells by G-CSF does, in fact, take place.
MATERIALS AND METHODS
The cancer cells used in this study were obtained from a 76-year-old man
with metastatic transitional cell carcinoma of the bladder who demonstrated
marked leukocytosis; his peripheral blood leukocyte count was 94.900 leuko-
cytes/mttr', and his serum G-CSF level was 103 pg/ml. Immunohistochemical
study of the cancer tissue obtained by biopsy with the use of a mAb against
recombinant human G-CSF was performed to identify the exact cell type
responsible for G-CSF production. Sections of the 5% formalin-fixed and
paraffin-embedded bladder tumor tissue specimen were studied by using the
avidin-biotin-peroxidase method. Mouse anti-human G-CSF mAb (KW341)
provided by Kyowa Hakkou Co, Ltd. (Tokyo, Japan) was used as the primary
antibody at a dilution of 1:50. To confirm the specificity of the immunohis-
tochemical study, tumor specimens from SCID mice implanted with CHO cells
transfected with human G-CSF cDNA (17) were examined as a positive
control, and as a negative control, mouse IgG was used as the primary antibody
instead of anti-G-CSF antibody.
The tumor tissue was minced into 1-mm3 pieces, placed on a culture flask
(25 cm2), and maintained in culture medium [RPMI 1640 and Eagle's MEM
diluted 1:1, supplemented with 10% heat-inactivated PCS, 1% insulin-trans-
ferine-sodium selenite medium supplement (Sigma, Japan), 100 (xg/ml strep
tomycin, and 100 international units/ml penicillin] in an atmosphere of 5%
CO, for additional culture.
Exponential growth of the cells was seen approximately 3 weeks after the
primary culture. Subsequently, the cells were subcultured with a split ratio of
1:10 every 4-6 days. G-CSF concentrations in the culture medium, on which
the cancer cells were grown exclusively, were measured by ELISA.
Both G-CSF and G-CSF receptor mRNA expression on the cultured cancer
cells were studied with the use of the RT-PCR method. Total RNA samples
were purified from the cultured cancer cells by the acid guanidine phenol-
chloroform method (18). The respective RNA (5 /ig) samples were converted
into cDNA with the use of oligo(dT) primers and reverse transcriptase (code
8089SA; Life Technologies) diluted with H2O to obtain 100 fj.1of the cDNA
preparation. Five-fil samples were subjected to the following PCR: («)the
ß-actin-specific fragment was detected by PCR (20 cycles at 94°Cfor 1 min,
65°C for 1 min, and 72°C for 3 min) with primers 5'-GATATCGC-
CGCGTCGTCGTCGAC-3' (forward primer) and 5'-CAGGAAGGAAG-
GCTGGAAGAGTGC-3' (reverse primer); (b) the G-CSF-specific 278-bp
fragment was detected by PCR (40 cycles at 94°Cfor 1 min, and 50°Cfor 1
min) with 5'-CTGTGTGCCACCTACAAG-3' (forward primer) and 5'-GC-
CATTCCCAGTTCTTCC-3' (reverse primer); and (c) the G-CSF receptor
a-chain 727-bp fragment was detected by PCR (35 cycles at 94°Cfor 1 min,
65°Cfor 1 min, and 72°Cfor 1 min) with 5'-ACAGTCCTCACCCTGAT-
GACCT-3' (forward primer) and 5'-TGCCTCTTAAAGGCCTGAGCTA-3'
(reverse primer). In addition, as markers of other hematopoietic growth factors,
GM-CSF and GM-CSF receptor mRNA expression on the cultured cancer cells
were studied by RT-PCR; (d) the 441-bp GM-CSF-specific fragment was
detected by PCR (43 cycles at 94°Cfor 30 s, and 63°Cfor 1 min) with primers
5'-CTGGAGATGTGGCTGCAGAGCC-3' (forward primer) and 5'-TGCT-
3438
on July 8, 2017. © 1995 American Association for Cancer Research. cancerres.aacrjournals.org Downloaded from