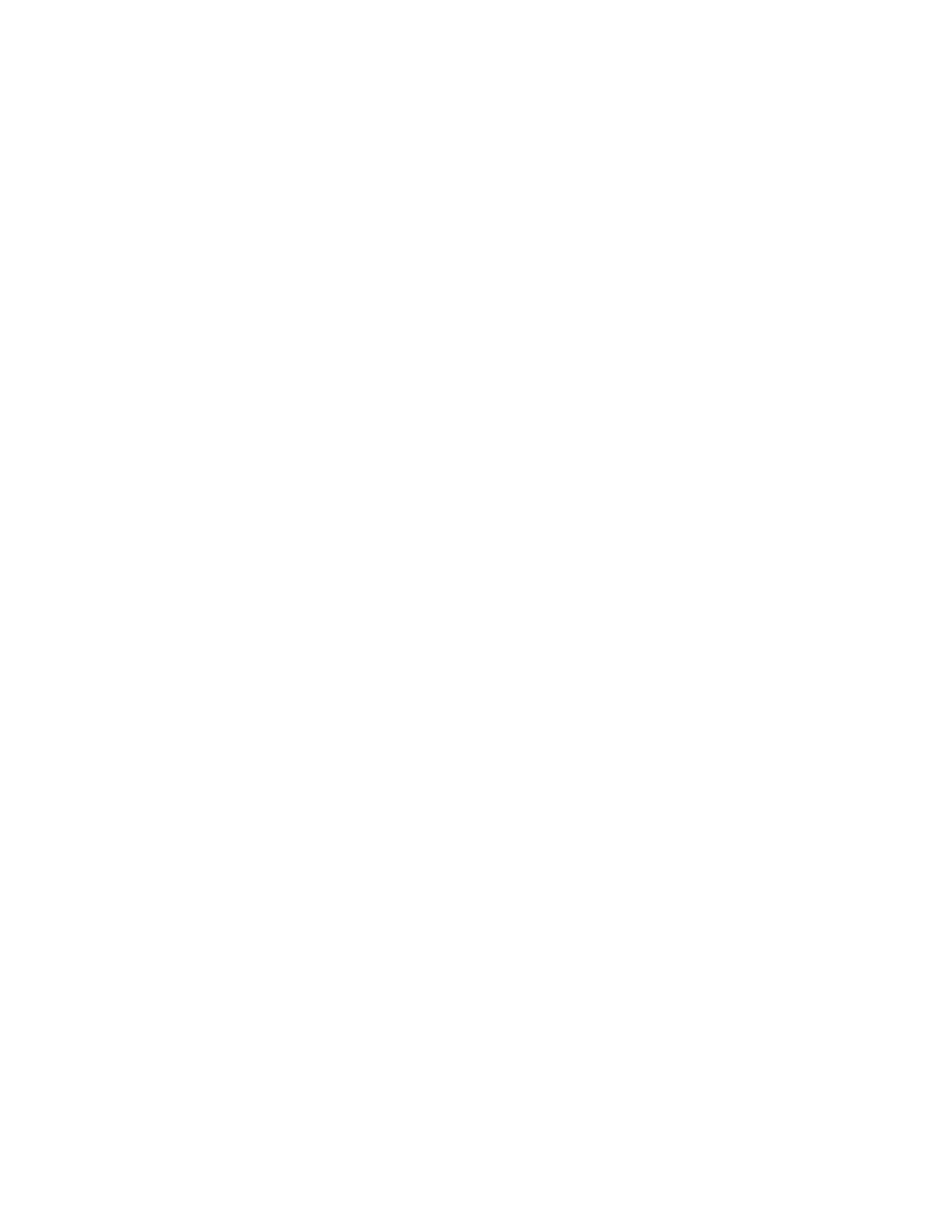
iv
Mike, Mohamed, Youjin, Ms. Maggie, Ms. Lori and Ms. Sze for the enjoyable group work and
stimulating discussions. Special appreciation to Dr. Shoraf Dadakhujaev and Dr. Susie Rosales
with whom I have enjoyed the many discussions we have had about science and life. I also
extend my thanks to Claude Veillette from Dr. Demetrick’s lab and to the members of Dr.
Riabowol’s lab: Arash, Subhash, Uma, Uyen, Yang and Ms. Donna for the ING3 (2A2)
antibody. Additionally, my gratitude goes to all members of the Translation lab at Tom Backer
Cancer Center, especially Mr. Brant Pohorelic for AQUA analysis and my best friend Alpana
Saxena. Thank you everyone for the friendship and generous support you have given me
throughout these years of my graduate study.
Moreover, I am deeply grateful and thankful to my family: my parents- Salma, and Ahmed;
my uncles Mohamed Naji Al-Amin, Mohamed Mohamed Al-Amin and Hamid Alwaly; my
brothers and sisters especially Mohamed Al-Husain and Zainab, for their unconditional love,
continuous care and constant support in every aspect of my life. Many thanks go, in particular, to
my husband, Abdullah, for his love and support, and also to my baby girl, Lama, for simply
being there.
I also must mention my indebtedness to my family members, friends and teachers in Canada
and back home in Saudi Arabia specifically Dr. Mohamed Basalamah from UQU and all
laboratory members at the Armed Forces Hospital, particularly Dr. Yousef Saleem. Last but not
least, I gratefully acknowledge financial support from the Ministry of Higher Education in the
Kingdom of Saudi Arabia and from the Royal Embassy of Saudi Arabia Cultural Bureau in
Canada.