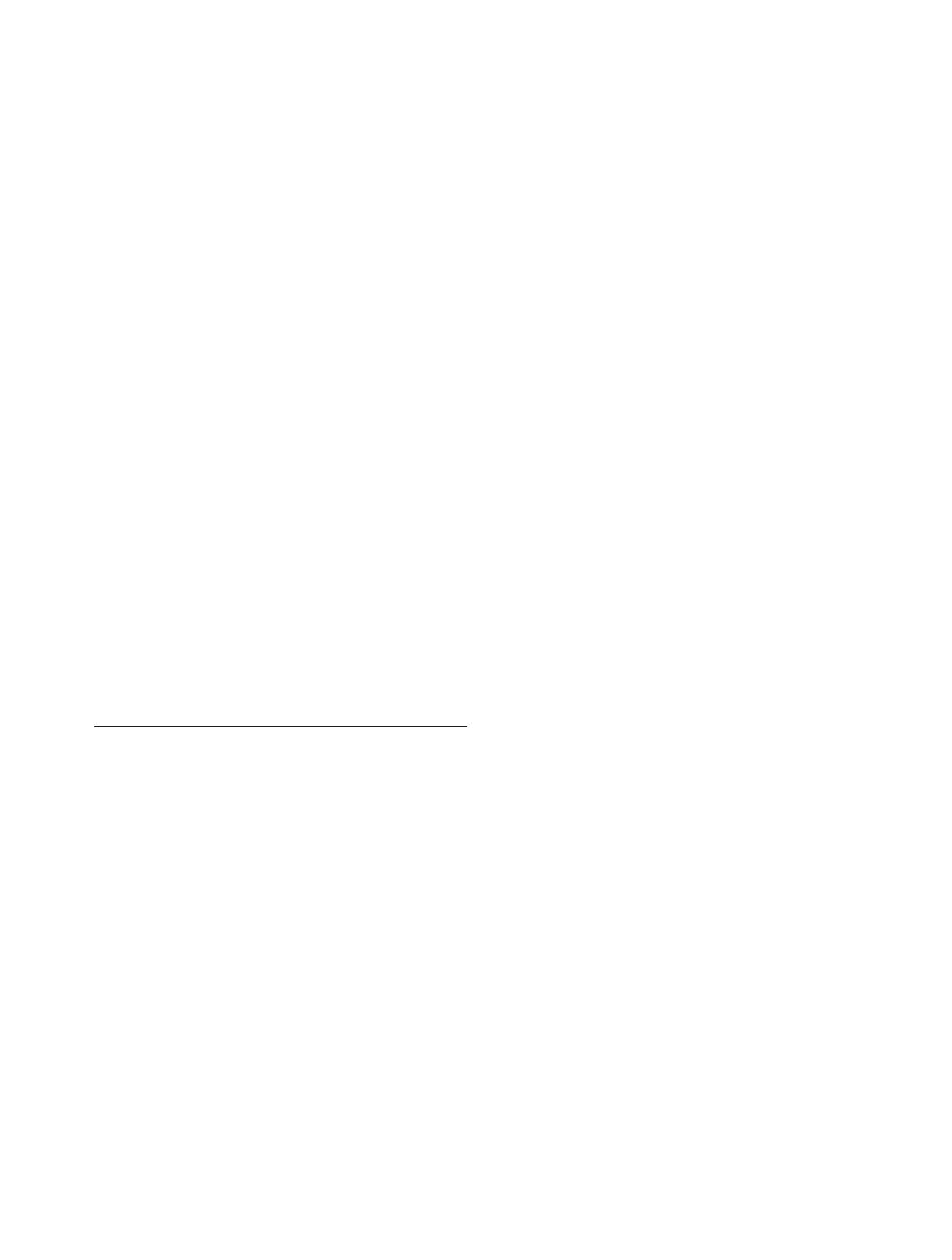
with an SH2 domain, binds to SHP-1, possibly recruiting phospho-
tyrosine-containing proteins for dephosphorylation by SHP-1.24
SHP-1–mediated dephosphorylation is also involved in delivery of
the Fas apoptosis signal in lymphoid cells.25 SHP-1 is positively
involved in epidermal growth factor (EGF) and interferon-␥–
induced signal transducer and activator of transcription (STAT)
activation in HeLa cells.26 In macrophages, SHP-1 also selectively
regulates the tyrosine phosphorylation of Stat1 and Jak1 while
leaving Tyk2 and Stat2 unaffected.27 In contrast, Keilhack et al28
have described the binding of SHP-1 to the EGF receptor, leading
to dephosphorylation of the receptor and attenuation of receptor
signaling. In the motheaten mouse, Fc␥RIIB signaling is deficient,
suggesting a role of SHP-1 in control of ITIM function.29 In
contrast, Ono et al 30,31 have shown that inhibitory signaling by
Fc␥RIIB does not require SHP-1 but involves the 5⬘inositol phos-
phatase SHIP. Data from Sharlow et al32 have indicated that
SHP-1 acts as a negative regulator of erythropoietin-induced
differentiation of normal erythroid progenitor cells, preventing
their premature commitment to terminal differentiation. Hence
it has been suggested that tyrosine phosphatases exert both
positive and negative regulation of specific signals and integrate
these signals temporally and spatially within the cell.
To investigate the role played by SHP-1 in phagocytic signal
transduction, we overexpressed wild-type SHP-1 in J774A.1 cells,
using a recombinant vaccinia virus expression system. SHP-1
overexpression led to a complete abrogation of phagocytosis of
sensitized sheep red blood cells (sRBCs) by J774A.1 cells. This is
the first evidence that SHP-1, a tyrosine phosphatase, regulates Fc␥
receptor–mediated phagocytosis. Most notably, SHP-1 associated
with the CBL adapter protein, and this association led to loss of
CBL phosphorylation and the suppression of Rac. These data
support a role for SHP-1 in the control of phagocytosis and suggest
that CBL dephosphorylation mediates, at least in part, negative
control of Fc␥R-dependent phagocytosis.
Materials and methods
Antibodies
Anti-CBL (SC170) and anti-CRKL antibodies (SC-319) were obtained from
Santa Cruz Biotechnology, (Santa Cruz, CA). Plasmids encoding enhanced green
fluorescent protein (EGFP)–tagged SYK kinase and Fc␥RIIA were prepared by
standard subcloning methods in pEGFP and pcDNA3.1, respectively. DNA
constructs encoding SHP-1, SHP-2, catalytically dead SHP-1 (C453S), or
catalytically dead SHP-2 (C486S) were subcloned into the pcDNA3.1 vector.
Anti-SYK antibody was provided by Dr Tamara Hurley (Salk Institute, San
Diego, CA), and anti–SHP-1 antibody was generated in our laboratory. For
detection of Fc␥RIIA expression in COS7 cells, we used an allophycocyanin
(APC) conjugate anti-CD32 monoclonal antibody (FL18.26).
Cell lines and vaccinia virus expression system
J774A.1, a macrophagelike cell line, was obtained from ATCC (Manassas,
VA; catalog no. 67-TIB).The cells were grown in Dulbecco Modified Eagle
Medium (DMEM) containing 10% fetal calf serum (FCS). Recombinant
vaccinia virus vectors were provided by Dr Bernard Moss (Bethesda, MD).
The dominant-negative SYK vaccinia construct (encoding the N terminus
of SYK, residues 1-255) was provided by Dr A. Scharenberg, as previously
described.33 Recombinant vaccinia viruses containing SHP-1 and dominant-
negative SYK were prepared as described. Briefly, recombinant vaccinia
virus was propagated in 149B cells grown in RPMI containing 10% FCS.A
confluent culture of cells was infected with recombinant vaccinia virus at a
concentration of 0.5 plaque-forming units (pfu) per cell for 48 hours. The
cells were scraped in the same medium, pelleted down, and resuspended in
5 mL of 10 mM Tris-HCl pH 9. The cells were disintegrated by freezing in
liquid nitrogen and thawing at 37°C 3 times, after which the volume was
made up to 20 mL with 10 mM Tris-HCl pH 9 and the cells were subjected
to 40 strokes in a homogenizer. Nuclei and cell debris were separated from
the cell lysate by centrifugation at 1000 rpm for 5 minutes. The cell lysate
containing the recombinant vaccinia virus was then subjected to sonication
for 1 minute. The cell lysate was loaded on a cushion of 36% sucrose
solution and centrifuged at 13 000 rpm for 80 minutes at 4°Cinan
ultracentrifuge (Beckman, PaloAlto, CA) using an SW.28 rotor.Viral pellet
obtained at the bottom was suspended in 1 mLof 10 mM Tris-HCl pH 9 and
loaded on a sucrose gradient composed of 6.6 mL each of 40%, 36%, 32%,
28%, and 24% sucrose solutions made in 10 mM Tris-HCl pH 9 to be
centrifuged at 12 500 rpm for 50 minutes at 4°C in an ultracentrifuge
(Beckman) using an SW.28 rotor. A bluish white ring containing purified
virus was collected, diluted with 10 mM Tris-HCl pH 9, and recentrifuged
at 13 000 rpm for 60 minutes at 4°C in an ultracentrifuge (Beckman) using
an SW.28 rotor to pellet the virus down. Purified recombinant vaccinia virus
thus obtained was suspended in 10 mM Tris-HCl pH 9 and titered as
follows. An aliquot was used for making serial dilutions of the viral
suspension. These were used to infect a confluent lawn of 149B cells grown
in a 6-well plate for 2 hours at 37°C in 1 mL RPMI containing 10% FCS.
The medium was then replaced with 3 mL of fresh RPMI containing 10%
FCS. After 24 hours the medium was discarded and the plaques were
visualized by staining with crystal violet to determine the titer.
Preparation of recombinant vaccinia virus containing
catalytically inactive mutant
A construct (C2mSHP-1S453KT3) containing catalytically inactive SHP-1
mutant was kindly provided by Dr Taolin Yi. The insert was amplified by
polymerase chain reaction (PCR), using the following 5⬘and 3⬘primers:
CTCGTCGACAGGATGGTGAGGTGGTTTCAC and AGTCCCGGGAGAT-
CACTTCCTCTTGAGAGAA, respectively. The amplified product was sub-
cloned into PCR2.1 vector with a TA cloning kit (Invitrogen, San Diego, CA),
isolated by using SmaI and SalI restriction digest, and ligated to the recombinant
vaccinia vector pSC65. The construct C2pSC65 was used to make a recombinant
vaccinia virus using packaging cell line CV1 and the wild-type vaccinia virus.
Recombinant virus was purified from the wild-type virus by single-plaque
purification. It was amplified, purified, and titered as described above.
Phagocytic assays
J774A.1 cells were plated at a density of 2 ⫻105cells per well in a 12-well
plate (Costar, Corning, NY) overnight. Cells were infected with recombi-
nant vaccinia virus pSC65 or pSC65-SHP-1 at a density of 2 pfu/cell for 4
hours at 37°Cin5%CO
2. After 4 hours the medium was changed and the
cells were subjected to sRBCs coated with IgG at subagglutinating
concentration. The target-to-effector ratio was kept at 100:1. The cells were
scraped after 2 hours; cytospins were prepared, fixed, and stained with
Wright Giemsa stain (Dade,AG, Switzerland); and the slides were observed
under a microscope for rosette formation. The rest of the cells were
subjected to water shock to lyse the uningested sRBCs. The cells were
suspended in DMEM containing 20% FCS. The cells were spun down on
glass slides and fixed and stained with Wright Giemsa stain. A minimum of
150 cells were counted for each slide and the phagocytic index was
calculated as follows: phagocytic index (PI) ⫽number of sRBCs internal-
ized by 100 J774 cells counted in 10 random fields of slide. In the case of
the inhibitors the cells were subjected to treatment with the inhibitor at
different concentrations along with an appropriate dimethyl sulfoxide
(DMSO) control for 1 hour in DMEM with 10% FCS before the
phagocytosis assay was carried out as described previously.
-Galactosidase and rosette formation assays
As a control for effects of recombinant vaccinia virus, J774A.1 cells were
tested for equal viral load by quantitation of -galactosidase activity
measured with X-gal. The plasmid pSC65, used for cloning of dominant-
negative SYK, contains the gene -galactosidase, which cleaves X-gal to
give a color product that can be quantitated colorimetrically. In every
SHP-1 REGULATES PHAGOCYTOSIS 1853BLOOD, 1 SEPTEMBER 2002 䡠VOLUME 100, NUMBER 5
For personal use only.on July 8, 2017. by guest www.bloodjournal.orgFrom