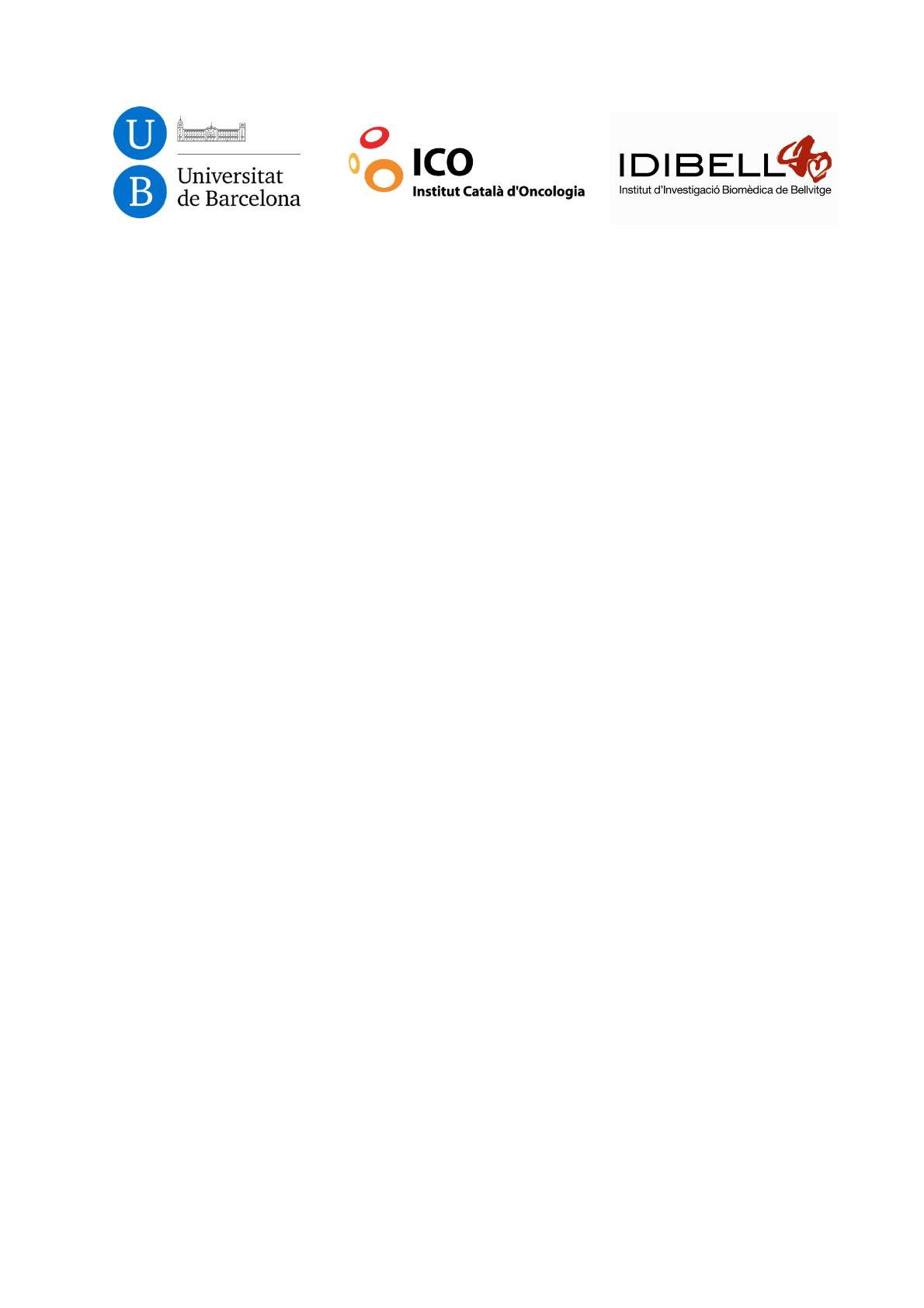
CARACTERIZACIÓN MOLECULAR Y BÚSQUEDA DE
CAUSAS GENÉTICAS DEL CÁNCER COLORRECTAL
HEREDITARIO NO POLIPÓSICO
Memoria presentada por
Fernando Bellido Molías
Para optar al grado de
Doctor por la Universidad de Barcelona
Tesis realizada bajo la dirección de la
Dra. Laura Valle Velasco
en el Instituto Catalán de Oncología del
Instituto de Investigación Biomédica de Bellvitge
(ICO-IDIBELL)
Tesis adscrita a la Facultad de Biología, Universidad de Barcelona (UB)
Programa de Doctorado en Genética
Tutor: Daniel Raúl Grinberg Vaisman
Laura Valle Velasco
Daniel Raúl Grinberg Vaisman
Fernando Bellido Molías
Barcelona, 2015