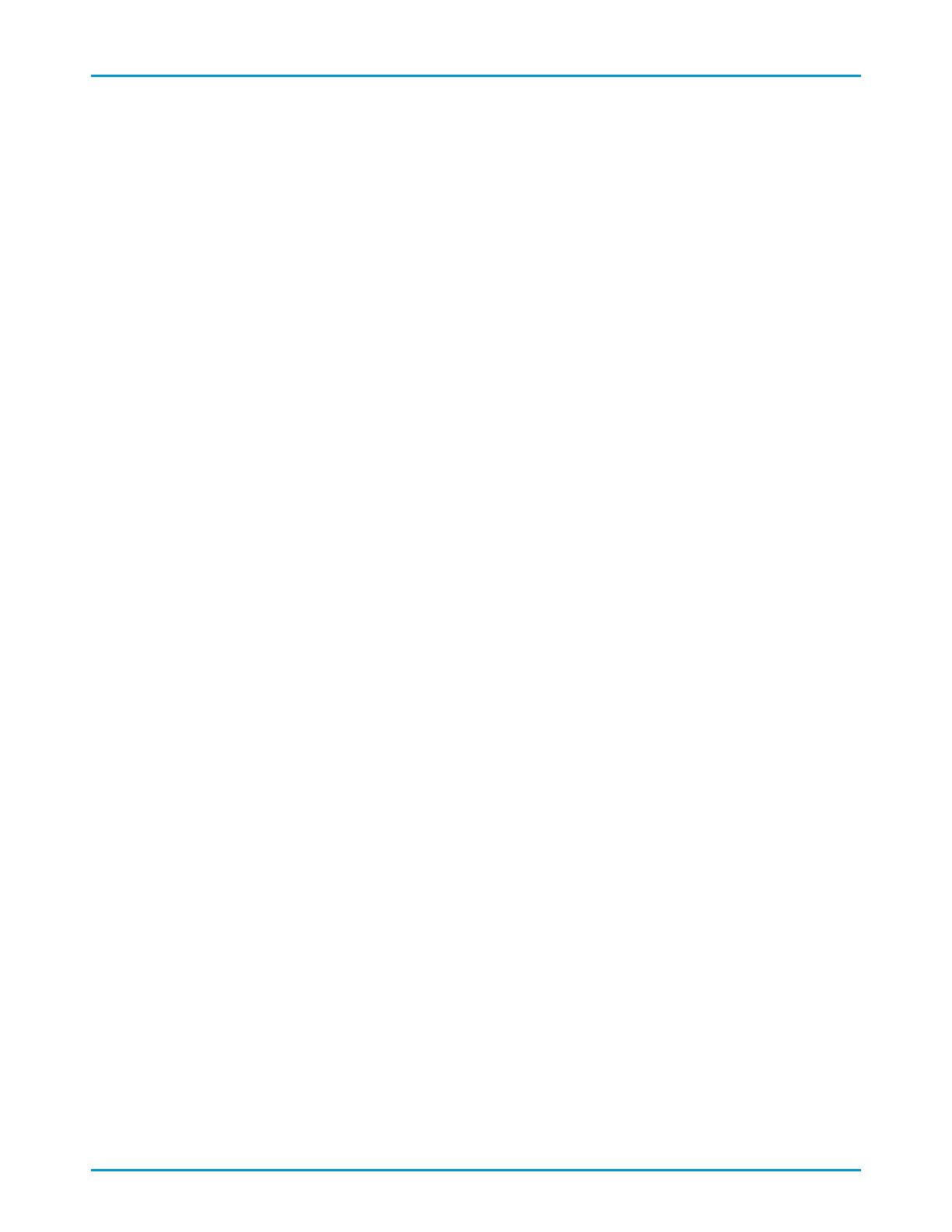
Theranostics 2012, 2(1)
http://www.thno.org
methods of conjugating the functional moieties to
nanoparticles; finally, we discuss strategies for opti-
mizing the nanoparticle surfaces for in vivo applica-
tions. We highlight the potential utility of magnetic
nanoparticle-based theranostic systems, which thus
far are shown to be suitable for clinical use.
Passive and active targeting
Most nanoparticles are expected to accumulate
in tumors due to the pathophysiologic characteristics
of tumor blood vessels. Delivery of nutrients to an
actively growing tumor with a volume greater than 2
mm3 becomes diffusion-limited, and new blood vessel
formation is required to supply nutrients and oxygen
[11]. The incomplete tumor vasculature results in
leaky vessels with enlarged gap junctions of 100 nm to
2 μm, depending on the tumor type, and macromol-
ecules easily access the tumor interstitium [12-14].
Tumors also have a compound retention time higher
than that of normal tissues because tumors lack a
well-defined lymphatic system [15,16]. These features
provide an enhanced permeability and retention
(EPR) effect, which constitutes an important mecha-
nism for the passive targeting and selective accumu-
lation of nanoparticles in the tumor interstitium. Dox-
il®, a poly(ethylene glycol)-coated (PEGylated) lipo-
somal system for doxorubicin (Dox) delivery, and
Abraxane®, albumin-bound paclitaxel nanoparticles
for the treatment of metastatic breast cancer, are rep-
resentative examples of US food and Drug Admin-
istration (FDA)-approved nanocarrier-based drugs for
cancer therapy. These agents circulate in the body
with a half-life about 100 times longer than that of free
anticancer drugs while simultaneously reducing sys-
temic toxicity [17-21].
However, passive targeting approaches suffer
from several limitations. Targeting cancer cells using
the EPR effect is not feasible in all tumors because the
degree of tumor vascularization and porosity of tu-
mor vessels can vary with the tumor type and status
[12,22]. In addition, cancer cells can display a reduced
number of specific interactions that lead to internali-
zation of nanoparticles. In addition to preventing in-
teractions between nanoparticles and opsonins,
PEGylated surfaces can also reduce interactions be-
tween nanoparticles and cell surfaces [23-26]. The lack
of control can lead to drug expulsion and induce
cancer cells to develop resistance toward a variety of
drugs (multiple drug resistance, MDR), which inevi-
tably reduces any therapeutic effects [27]. One ap-
proach to overcoming these limitations is to attach
targeting moieties to the nanoparticle surfaces. Na-
noparticles that present targeting moieties can bind to
target cells through ligand-receptor interactions that
induce receptor-mediated endocytosis and drug re-
lease inside the cell. Efficient binding and internaliza-
tion requires that receptors are expressed exclusively
on target cancer cells (104–105 copies per cell) relative
to normal cells, and expression should be homoge-
nous across all targeted cells [28]. This delivery strat-
egy achieves a high targeting specificity and delivery
efficiency, while avoiding nonspecific binding and the
MDR efflux mechanism [29]. At present, several tar-
geted delivery systems are under clinical trials, such
as transferrin receptor targeted cytotoxic plati-
num-based oxaliplatin in a liposome (MBP-426),
transferrin receptor targeted cyclodextrin-containing
nanoparticles with siRNA payload (CALAA-01), or
prostate-specific membrane antigen (PSMA) targeted
polymeric nanoparticles containing docetaxel
(BIND-014). Table 1 lists the nanoparticle-based drugs
that are approved or under clinical development.
Although ligand-mediated targeting technologies
have not yet made a considerable clinical impact on
human health, it will soon be feasible to develop tar-
geted nanoparticle candidates for clinical translation
[30].
Multifunctional nanoparticles for targeted im-
aging and therapy
The multifunctional properties of nanoparticles
convey unique advantages for the cancer-specific de-
livery of imaging and therapeutic agents [42]. Several
ligands with therapeutic, diagnostic, or barri-
er-avoiding properties can be incorporated across the
large nanoparticle surface area in a single nanoparti-
cle system. Multivalent targeting significantly in-
creases the binding affinity of a particle toward a tar-
get cell [43]. Magnetic iron oxide nanoparticles have
been shown to be suitable for use as theranostic
agents by employing their intrinsic diagnostic capa-
bilities in the context of MRI applications. Surface
modifications may be easily introduced through con-
jugation with targeting moieties (e.g., antibodies,
peptides, small molecules, or aptamers), fluorescence
dyes, genes, or drugs to provide multimodal func-
tionalities [44-47]. In the following section, multifunc-
tional nanoparticle systems that feature a variety of
targeting moieties for in vitro and/or in vivo cancer
imaging and therapy, including magnetic nanoparti-
cles, will be discussed.
2. Types of targeting moieties
Targeting moieties are classified as proteins
(mainly antibodies and their fragments), peptides,
nucleic acids (aptamers), small molecules, or others
(vitamins or carbohydrates). Although monoclonal