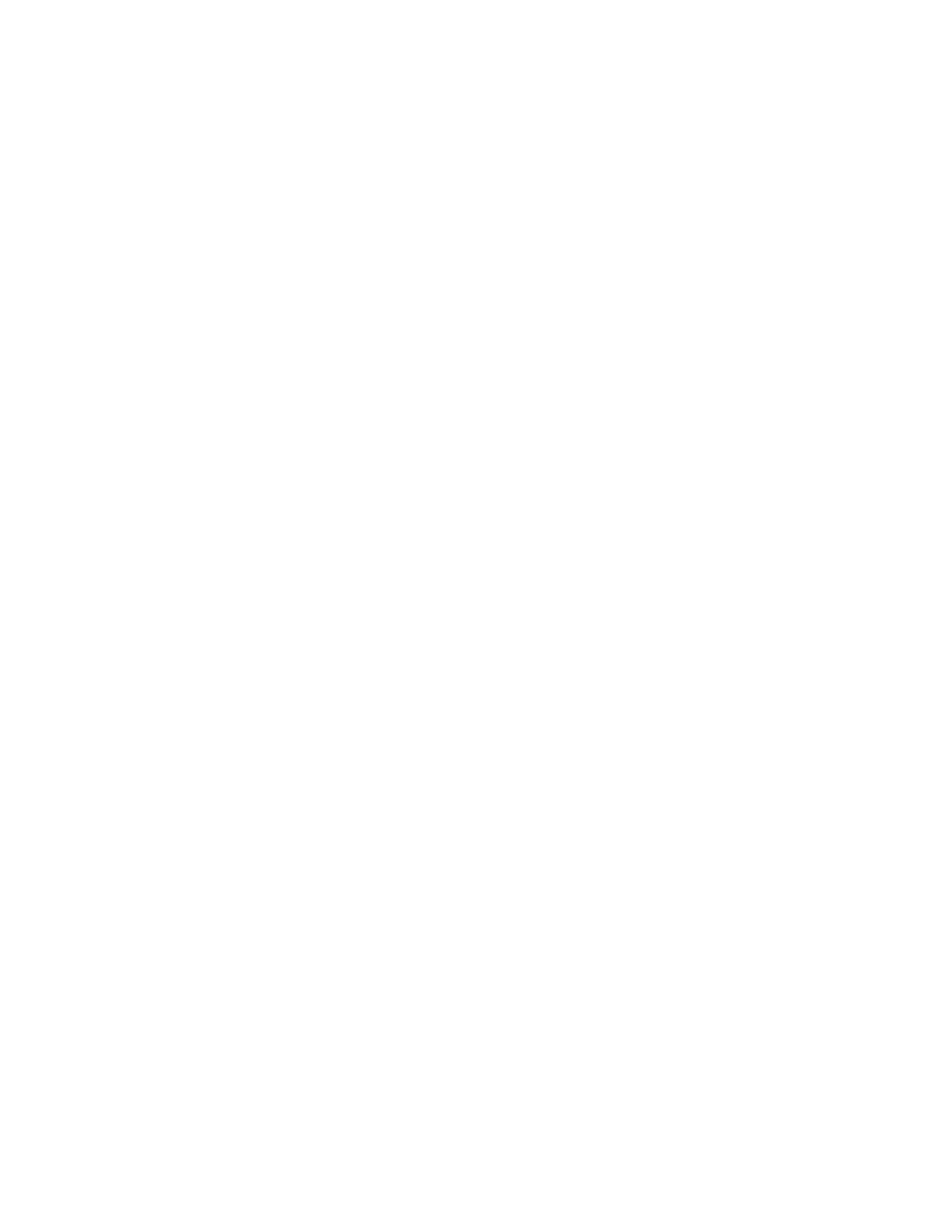
v
Table of Contents
Approval Page ..................................................................................................................... ii
Abstract ............................................................................................................................... ii
Acknowledgements ............................................................................................................ iii
Table of Contents .................................................................................................................v
List of Tables ................................................................................................................... viii
List of Figures and Illustrations ......................................................................................... ix
List of Symbols, Abbreviations and Nomenclature ........................................................... xi
CHAPTER ONE: INTRODUCTION ..................................................................................1
1.1 HIV and AIDS ...........................................................................................................2
1.1.1 HIV-1 Cell Infection ..........................................................................................5
1.2 The HIV-1 Nef Protein ..............................................................................................9
1.2.1 Functions of the Nef Protein ............................................................................10
1.2.2 Effect on Pathogenesis ....................................................................................10
1.3 HIV-1 Pathogenesis and the Gastrointestinal Tract .................................................12
1.3.1 HIV-1 and GI Barrier Dysfunction ..................................................................12
1.3.2 Nef and the GI Tract ........................................................................................14
1.4 The GI Tract .............................................................................................................20
1.4.1 Mucosal Epithelial Layer ................................................................................22
1.4.2 Tight Junctions ................................................................................................23
1.5 The Co Culture Barrier Model .................................................................................24
1.6 Summary ..................................................................................................................26
1.7 Research Hypothesis and Aims ...............................................................................27
CHAPTER TWO: EXPERIMENT METHODS AND MATERIALS ..............................28
2.1 Cell Culture Lines and Bacteria ...............................................................................28
2.1.1 Tissue Culture Cells ........................................................................................28
2.1.2 Bacteria ............................................................................................................30
2.2 Sindbis Virus Expression .........................................................................................31
2.2.1 Production of SINrep5-EGFP ..........................................................................31
2.2.2 Viral Titer and Epithelial Cell Infection ..........................................................32
2.3 pcDNA3 Expression Vector: ...................................................................................33
2.3.1 pcDNA3 Constructs .........................................................................................33
2.3.1.1 PCR Amplification of Nef .....................................................................33
2.3.1.2 Subcloning .............................................................................................35
2.4 Transfection Reactions ............................................................................................37
2.4.1 Lipofection ......................................................................................................37
2.4.2 Neon Electroporation System ..........................................................................38
2.5 Co-Culture System ...................................................................................................41
2.5.1 Indirect Toxicity ..............................................................................................43
2.6 Transepithelial Electrical Resistance Studies ..........................................................44
2.6.1 Direct Toxicity .................................................................................................44
2.6.2 Indirect Toxicity ..............................................................................................44
2.7 Real-time Quantitative PCR (RT-PCR) ...................................................................45
2.7.1 Nucleic Acid Isolation .....................................................................................45