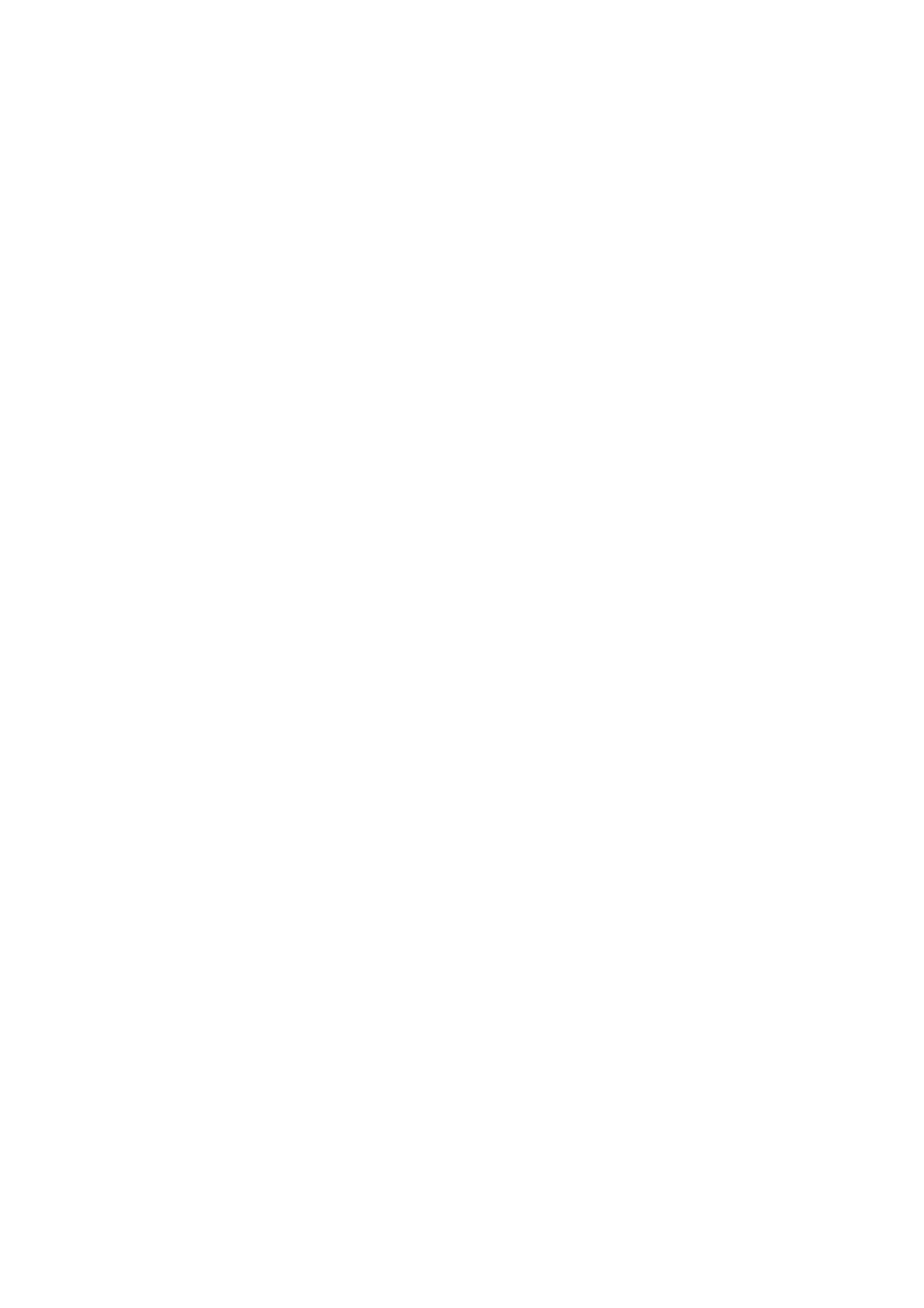
Departament de Biologia Cel·lular, de Fisiologia i d’Immunologia
Universitat Autònoma de Barcelona
Potential New Therapeutic Agents:
Effects on HIV Replication and Viral Escape
Gemma Moncunill Piñas
Laboratori de retrovirologia
Fundació irsiCaixa
Hospital Universitari Germans Trias i Pujol
Memòria de la tesi presentada per obtenir el grau de Doctora en Immunologia per la
Universitat Autònoma de Barcelona
Bellaterra, 2 de desembre del 2008
Director: Dr. José A. Esté
Tutora: Dra. Paz Martínez
Amb el suport del Departament d’Educació i Universitats de la Generalitat de Catalunya