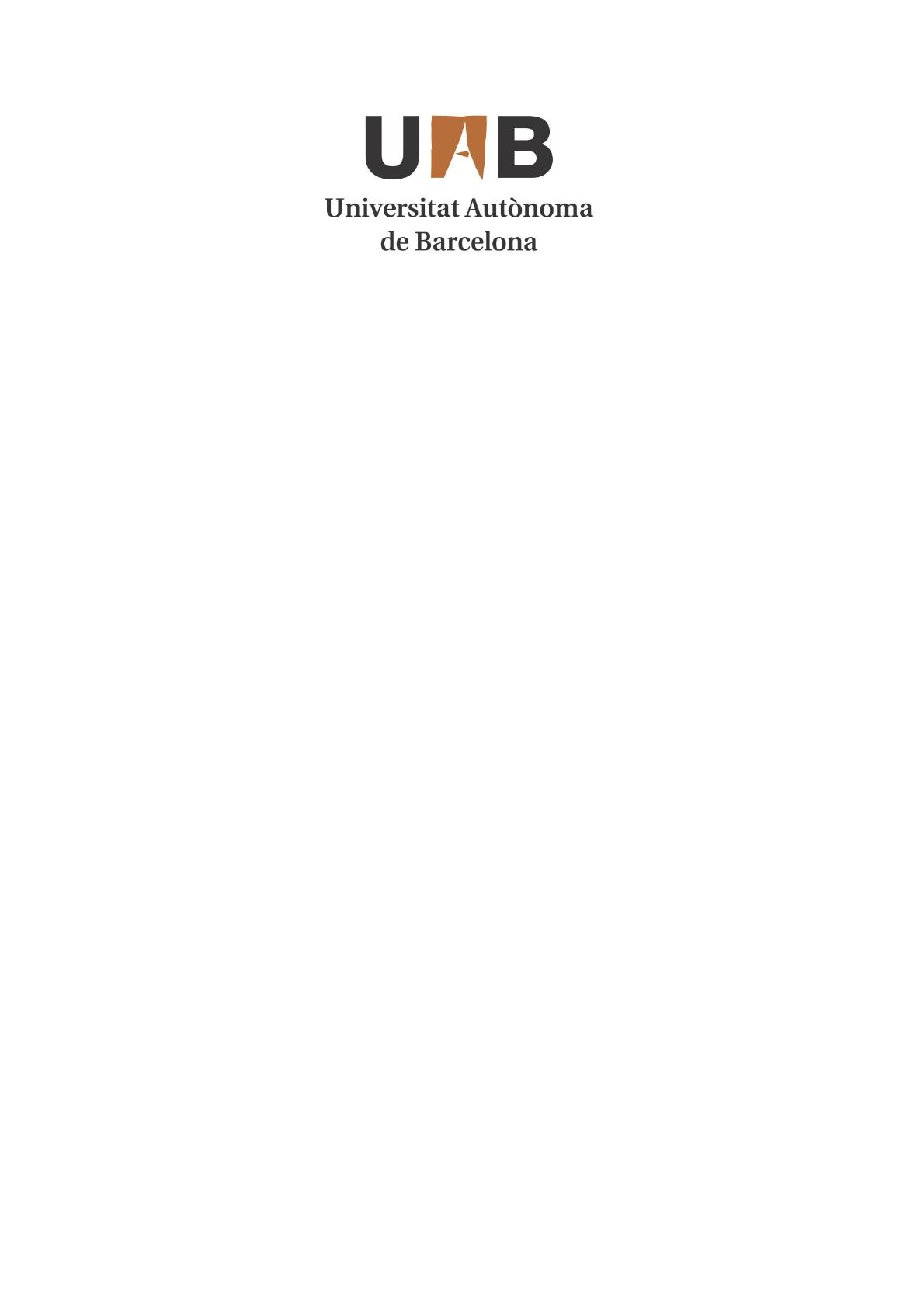
3
ScalinguprecombinantBCGbased
HIVvaccinedevelopment.Lessons
learned
TesipresentadaperenNarcísSaubiRocaperoptaral
TítoldeDoctorenBioquímica,BiologiaMoleculariBiomedicina
perlaUniversitatAutònomadeBarcelona
Doctorand:
NarcísSaubiRoca,
Director:Tutor:
Dr.JoanJosephMunné,Dr.JaumeFarrésVicén
InvestigadordelGrupdeRecercadelVIH
delaFundacióClínicperlaRecerca
Biomèdica‐HospitalClínicdeBarcelona
Catedràticd’UniversitatdelDptde
Bioquímica,BiologiaMoleculari
BiomedicinadelaUniversitatAutònomade
Barcelona