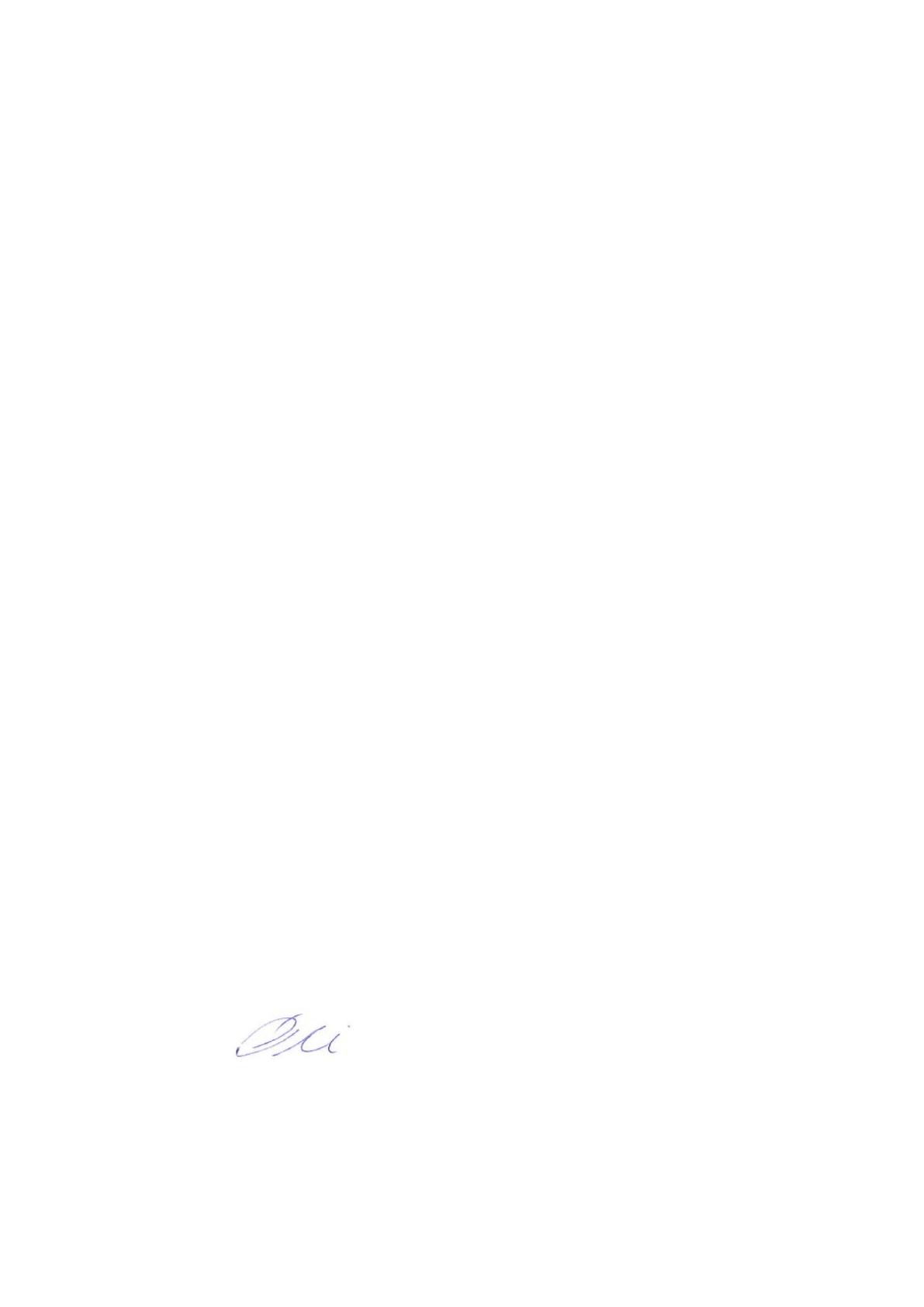
La Dra. TUIJA KEKARAINEN, investigadora del Centre Recerca en Sanitat Animal
(CReSA), i el Dr. JOAQUIM SEGALÉS COMA, professor titular del Departament de
Sanitat i d’Anatomia Animals de la Facultat de Veterinària de la Universitat Autònoma de
Barcelona i investigador del CReSA,
Certifiquen:
Que la memòria de tesi doctoral titulada “Torque teno sus viruses: pathogenesis in co-
infection with Porcine circovirus type 2 and humoral immune responses during natural
infection of pigs” presentada per David Nieto Blanco per l’obtenció del grau de Doctor en
Medicina i Sanitat Animals, s’ha realitzat sota la nostra direcció al Centre de Recerca en
Sanitat Animal (CReSA).
I per tal de que consti, als efectes oportuns, signem el present certificat a Bellaterra, a 16 de
Setembre de 2015.
Els directors
Dra. Tuija Kekarainen Dr. Joaquim Segalés i Coma