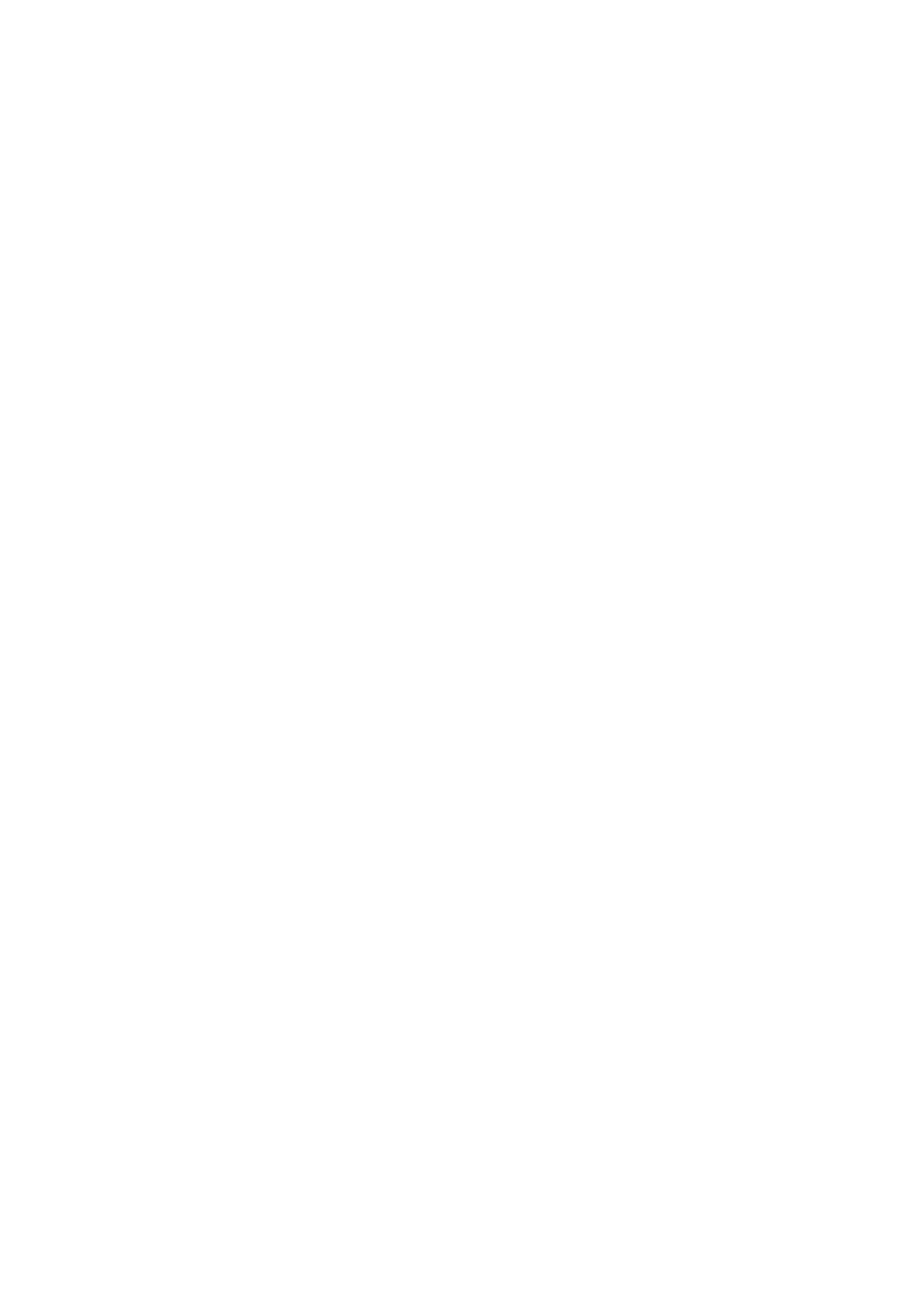
Department of Cellular Biology, Physiology and
Immunology, Faculty of Medicine, Universitat Autònoma
de Barcelona
Targeting a major HIV-1 vulnerability
region: the gp41 Membrane Proximal
External Region
Balance between neutralizing and non-neutralizing
antibodies and implications for vaccine design
Luis Manuel Molinos Albert
Institut de Recerca de la Sida IrsiCaixa & Institut d´Investigació en
Ciències de la Salut Germans Trias I Pujol (IGTP)
Hospital Universitari Germans Trias I Pujol
Doctoral thesis to obtain the PhD degree in Immunology of the Universitat
Autònoma de Barcelona, June 2016
Directors: Dr. Julià Blanco Arbués
Dr. Jorge Carrillo Molina