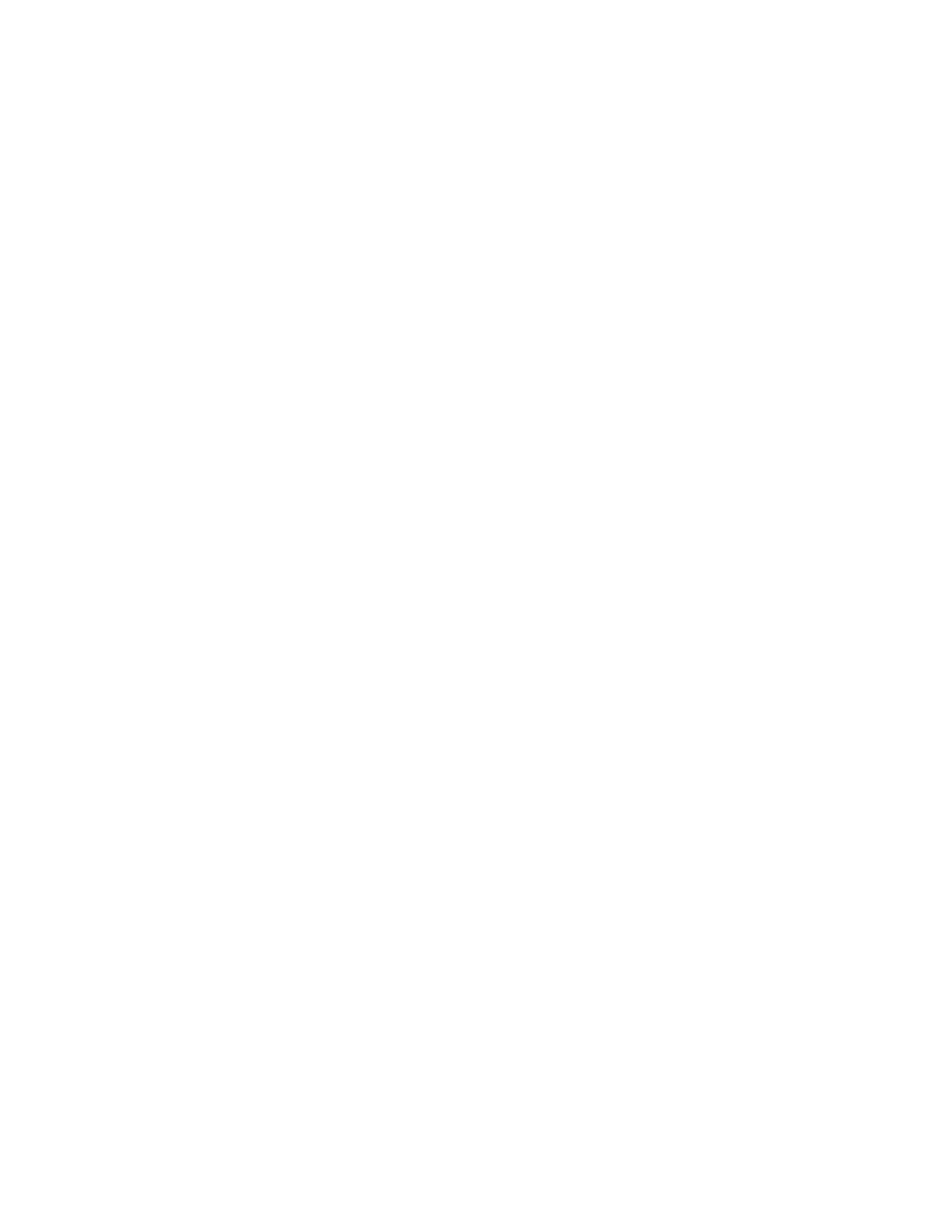
arXiv:1204.4789v2 [astro-ph.EP] 25 Apr 2012
DRAFT VERSION FEBRUARY 8, 2013
Preprint typeset using L
A
TEX style emulateapj v. 5/2/11
A NEW TYPE OF AMBIGUITY IN THE PLANET AND BINARY INTERPRETATIONS OF CENTRAL PERTURBATIONS
OF HIGH-MAGNIFICATION GRAVITATIONAL MICROLENSING EVENTS
J.-Y. CHOI1, I.-G. SHIN1, C. HAN1,G1,S, A. UDALSKIO01,G1, T. SUMIM01,G2, A. GOULDu01,G3, V. BOZZA 702,722,G4, M. DOMINIK 703,⋆,G4, P.
FOUQUÉP01,G5, K. HORNE703,G6,
AND
M. K. SZYMA ´
NSKIO01, M. KUBIAKO01, I. SOSZY ´
NSKIO01, G. PIETRZY ´
NSKIO01,O02, R. POLESKIO01, K. ULACZYKO01, P.
PIETRUKOWICZO01, S. KOZŁOWSKIO01, J. SKOWRONu01, Ł. WYRZYKOWSKIO01,O03
(THE OGLE COLLABORATION),
F. ABEM02, D.P. BENNETTM03, I.A. BONDM04, C.S. BOTZLERM05, P. CHOTEM06, M. FREEMANM05, A. FUKUIM07, K. FURUSAWAM02, Y.
ITOWM02, S. KOBARAM02, C.H. LINGM04 K. MASUDAM02, Y. MATSUBARAM02, N. MIYAKEM02, Y. MURAKIM02, K. OHMORIM02, K.
OHNISHIM08, N.J. RATTENBURYM05, TO. SAITOM10, D.J. SULLIVANM06, D. SUZUKIM01, K. SUZUKIM02, W.L. SWEATMANM04, S.
TAKINOM02, P.J. TRISTRAMM06, K. WADAM01, P.C.M. YOCKM05
(THE MOA COLLABORATION),
D.M. BRAMICHR04, C. SNODGRASSR06, I.A. STEELER05, R.A. STREETR02, Y. TSAPRASR02,R03
(THE ROBONET COLLABORATION),
K.A. ALSUBAI701, P. BROWNE703, M.J. BURGDORF704,705, S. CALCHI NOVATI702,706, P. DODDS703, S. DREIZLER707, X.-S. FANG708, F.
GRUNDAHL709, C.-H. GU708, S. HARDIS710, K. HARPSØE 710,711, T.C. HINSE710,712,713, A. HORNSTRUP714, M. HUNDERTMARK703,707, J.
JESSEN-HANSEN709, U.G. JØRGENSEN710,711, N. KAINS715, E. KERINS716, C. LIEBIG703, M. LUND709, M. LUNKKVIST709, L.
MANCINI717,718, M. MATHIASEN710, M.T. PENNY716,u01, S. RAHVAR719,728, D. RICCI720, G. SCARPETTA702,706,722, J. SKOTTFELT710, J.
SOUTHWORTH725, J. SURDEJ720, J. TREGLOAN-REED725, J. WAMBSGANSS726, O. WERTZ720
(THE MINDSTEPCONSORTIUM),
L. A. ALMEIDAu02, V. BATISTAu01, G. CHRISTIEu03, D.L. DEPOYu04, SUBO DONGu05, B.S. GAUDIu01, C. HENDERSONu01, F.
JABLONSKIu02, C.-U. LEE713, J. MCCORMICKu07, D. MCGREGORu01, D. MOORHOUSEu08, T. NATUSCHu03,u09, H. NGANu03, S.-Y. PARK1,
R.W. POGGEu01, T.-G. TANu10, G. THORNLEYu08, J.C. YEEu01
(THE µFUN COLLABORATION),
M.D. ALBROWP04, E. BACHELETP01, J.-P. BEAULIEUP03, S. BRILLANTP08, A. CASSANP03, A.A. COLEP05, E. CORRALESP03, C.
COUTURESP03, S. DIETERSP05, D. DOMINIS PRESTERP09, J. DONATOWICZP10, J. GREENHILLP05, D. KUBASP08,P03, J.-B. MARQUETTEP03,
J.W. MENZIESP02, K.C. SAHUP06, M. ZUB726
(THE PLANET COLLABORATION),
1Department of Physics, Institute for Astrophysics, Chungbuk National University, Cheongju 371-763, Korea
O01Warsaw University Observatory, Al. Ujazdowskie 4, 00-478 Warszawa, Poland
O02Universidad de Concepción, Departamento de Astronomia, Casilla 160–C, Concepción, Chile
O03Institute of Astronomy, University of Cambridge, Madingley Road, Cambridge CB3 0HA, United Kingdom
M01Department of Earth and Space Science, Osaka University, Osaka 560-0043, Japan
M02Solar-Terrestrial Environment Laboratory, Nagoya University, Nagoya, 464-8601, Japan
M03University of Notre Dame, Department of Physics, 225 Nieuwland Science Hall, Notre Dame, IN 46556-5670, USA
M04Institute of Information and Mathematical Sciences, Massey University, Private Bag 102-904, North Shore Mail Centre, Auckland, New Zealand
M05Department of Physics, University of Auckland, Private Bag 92-019, Auckland 1001, New Zealand
M06School of Chemical and Physical Sciences, Victoria University of Wellington, PO BOX 60, Wellington, New Zealand
M07Okayama Astrophysical Observatory, NAOJ, Okayama 719-0232, Japan
M08Nagano National College of Technology, Nagano 381-8550, Japan
M10Tokyo Metropolitan College of Aeronautics, Tokyo 116-8523, Japan
R02Las Cumbres Observatory Global Telescope Network, 6740B Cortona Dr, Goleta, CA 93117, USA
R03School of Physics and Astronomy, Queen Mary University of London, Mile End Road, London, E1 4NS
R04European Southern Observatory, Karl-Schwarzschild-Str. 2, 85748 Garching bei München, Germany
R05Astrophysics Research Institute, Liverpool John Moores University, Liverpool CH41 1LD, UK
R06Max Planck Institute for Solar System Research, Max-Planck-Str. 2, 37191 Katlenburg-Lindau, Germany
701Qatar Foundation, P.O. Box 5825, Doha, Qatar
702Università degli Studi di Salerno, Dipartimento di Fisica "E.R. Caianiello", Via Ponte Don Melillo, 84084 Fisciano (SA), Italy
703SUPA, University of St Andrews, School of Physics & Astronomy, North Haugh, St Andrews, KY16 9SS, United Kingdom
704Deutsches SOFIA Institut, Universität Stuttgart, Pfaffenwaldring 31, 70569 Stuttgart, Germany
705SOFIA Science Center, NASA Ames Research Center, Mail Stop N211-3, Moffett Field CA 94035, United States of America
706Istituto Internazionale per gli Alti Studi Scientifici (IIASS), Vietri Sul Mare (SA), Italy
707Institut für Astrophysik, Georg-August-Universität, Friedrich-Hund-Platz 1, 37077 Göttingen, Germany
708National Astronomical Observatories/Yunnan Observatory, Joint laboratory for Optical Astronomy, Chinese Academy of Sciences, Kunming 650011,
People’s Republic of China
709Department of Physics and Astronomy, Aarhus University, Ny Munkegade 120, 8000 Århus C, Denmark
710Niels Bohr Institute, University of Copenhagen, Juliane Maries vej 30, 2100 Copenhagen, Denmark
711Centre for Star and Planet Formation, Geological Museum, Øster Voldgade 5, 1350 Copenhagen, Denmark
712Armagh Observatory, College Hill, Armagh, BT61 9DG, Northern Ireland, United Kingdom
713Korea Astronomy and Space Science Institute, 776 Daedukdae-ro, Yuseong-gu, Daejeon 305-348, Republic of Korea
714Danmarks Tekniske Universitet, Institut for Rumforskning og -teknologi, Juliane Maries Vej 30, 2100 København, Denmark
715ESO Headquarters, Karl-Schwarzschild-Str. 2, 85748 Garching bei München, Germany
716Jodrell Bank Centre for Astrophysics, University of Manchester, Oxford Road,Manchester, M13 9PL, UK
717Max Planck Institute for Astronomy, KÄonigstuhl 17, 69117 Heidelberg, Germany