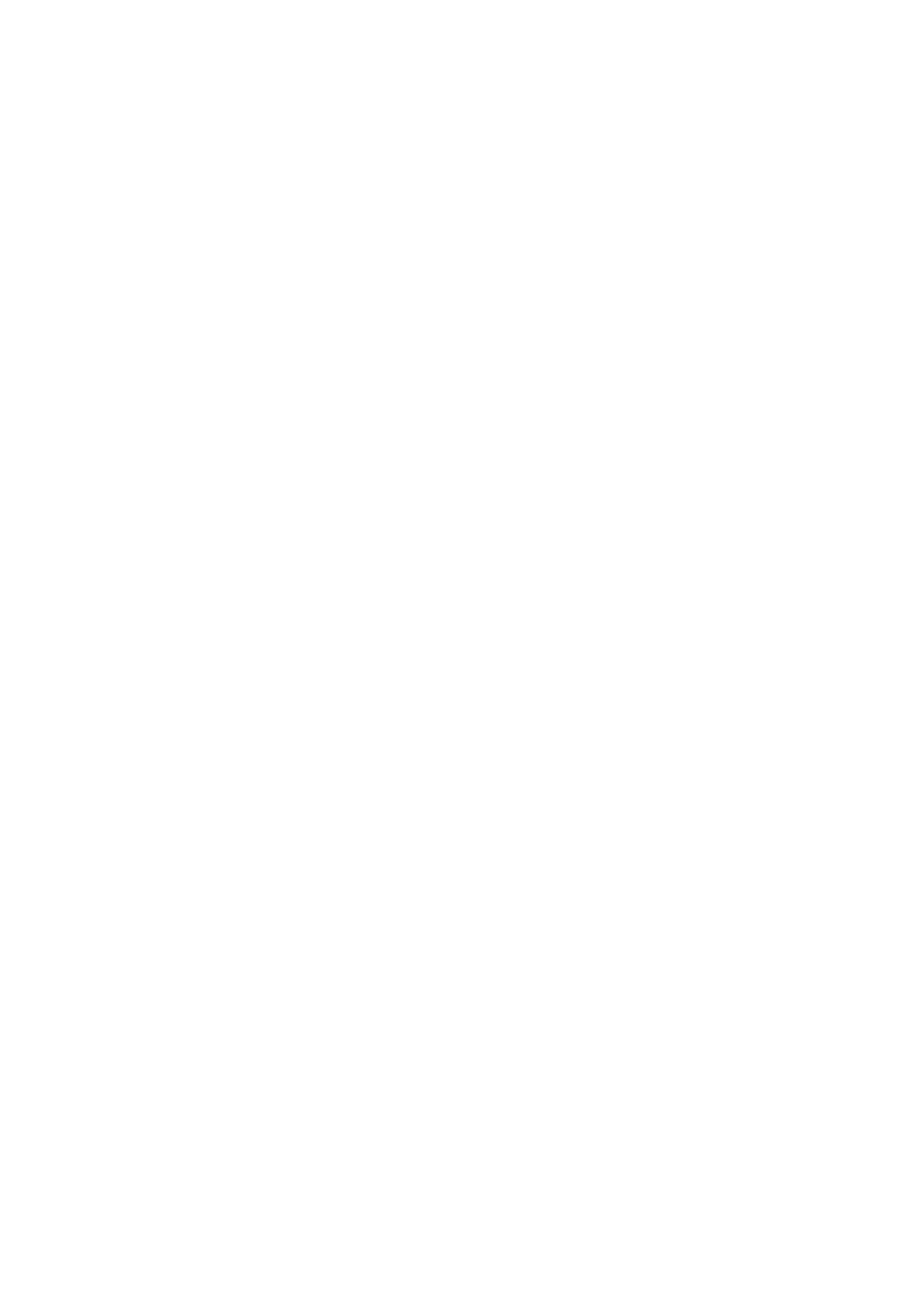
III
Thanks to Dr. Stamatiki Roussi and Dr. Charlotte Foltzer-Jourdainne for her guidance,
friendship, kindless and encouraged me at the beginning of my doctoral studies. Thanks
also to Dr. Souad Bousserouel for their critical comments.
An special thanks to my colleague and friend Virginie Lamy for her friendship, her
unconditional support and encourage, a wonderful colleague and a brilliant professional
who shared with me during these three years all the difficulties that brings a thesis, but also
taught me the beauty of French and alsaciene cultures.
Thanks to my superiors in the University of Antioquia, and my colleagues, especially
Prof. Gina Montoya and Prof. Claudia Velásquez for their support before and during my
doctoral studies, for the encouragement and critical comments, and the most important, for
her friendship.
I am grateful to my family who accompanied me in these long three years, my mother
that gave me their advices and for all their prayers, for
guide me in the right direction during the development of this thesis work, in this foreign
city, far from my home. Thanks to my brother and my sisters that made me feel that
despite of the distance the same blood joint us.
I am grateful to my husband Libardo, my charming love, my friend, the man who
supported and waited me day and night during these three years, the man who encouraged
me and believe on me in the good and the not so good moments, for his motivation every
day spite of distance. My first reason that make me wake-up every day to work enthusiastic
until the end of my thesis.
Finally, thanks to God and life for give me the opportunity to study in France with all
the facilities and resources to do my best, by the opportunity to see new cultures, countries
and to learn how big our world is; for this wonderful experience that made me better
human being, wife, daughter, sister and professional.