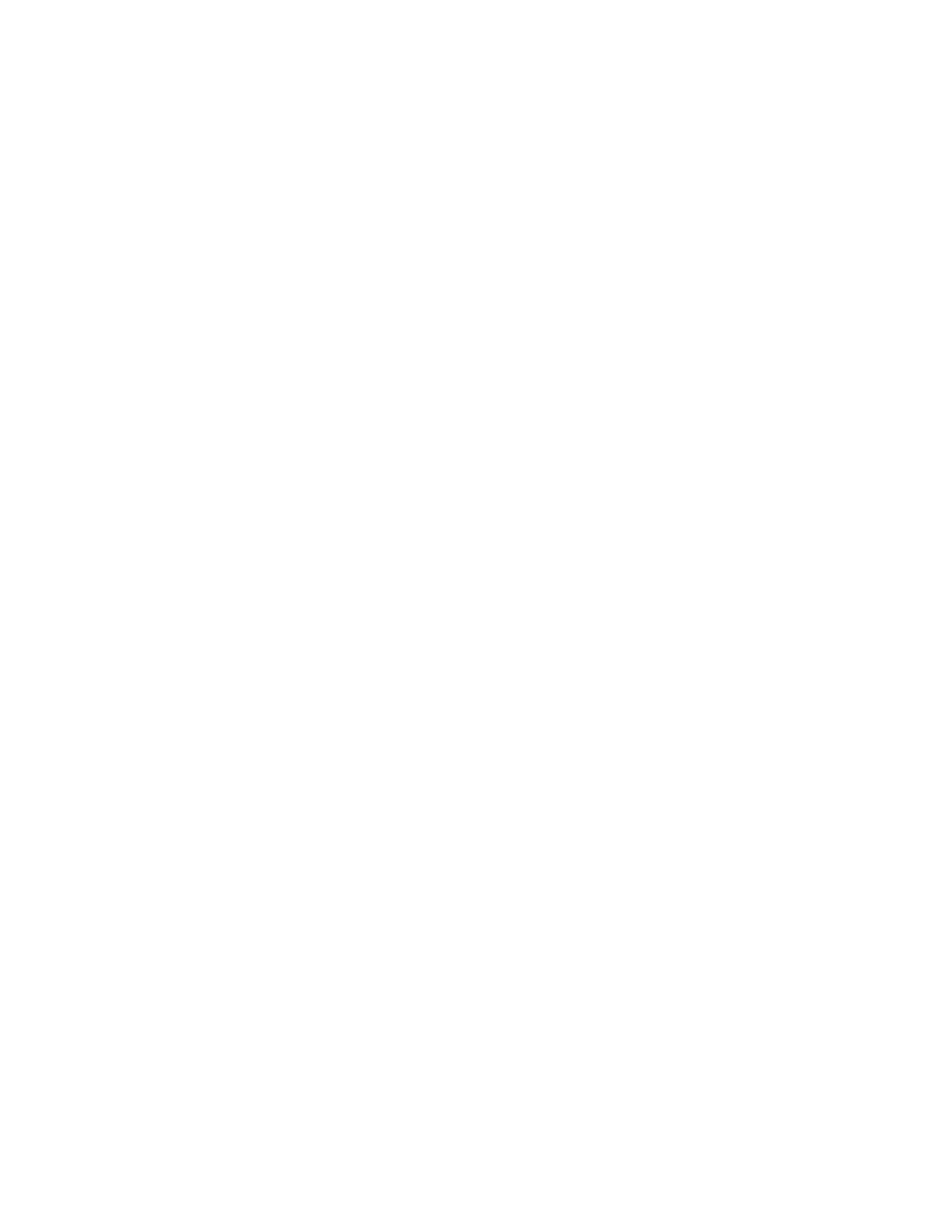
iii
Résumé
La récepti est un point crucial en reproduction. Il
éparent le
communique avec
laboratoire, portant sur le système IL (interleukine) 1, la hCG (hormone
gonadotrophine chorionique humaine) et , nous avons
étudié le rôle de ces deux facteurs embryonnaires précoces, dans
naire est
strictement fondamental et représente la base pour une meilleure compréhension
. Pour se faire, des approches in vitro et in vivo
ont été utilisées. est ciblée de
façon originale par la hCG. Celle-
déséquilibre pro-
ble modérer certains
s cibles par effet de coopération. Nos travaux démontrent
lien avec la présence du chef de file des facteurs embryonnaires, la hCG.