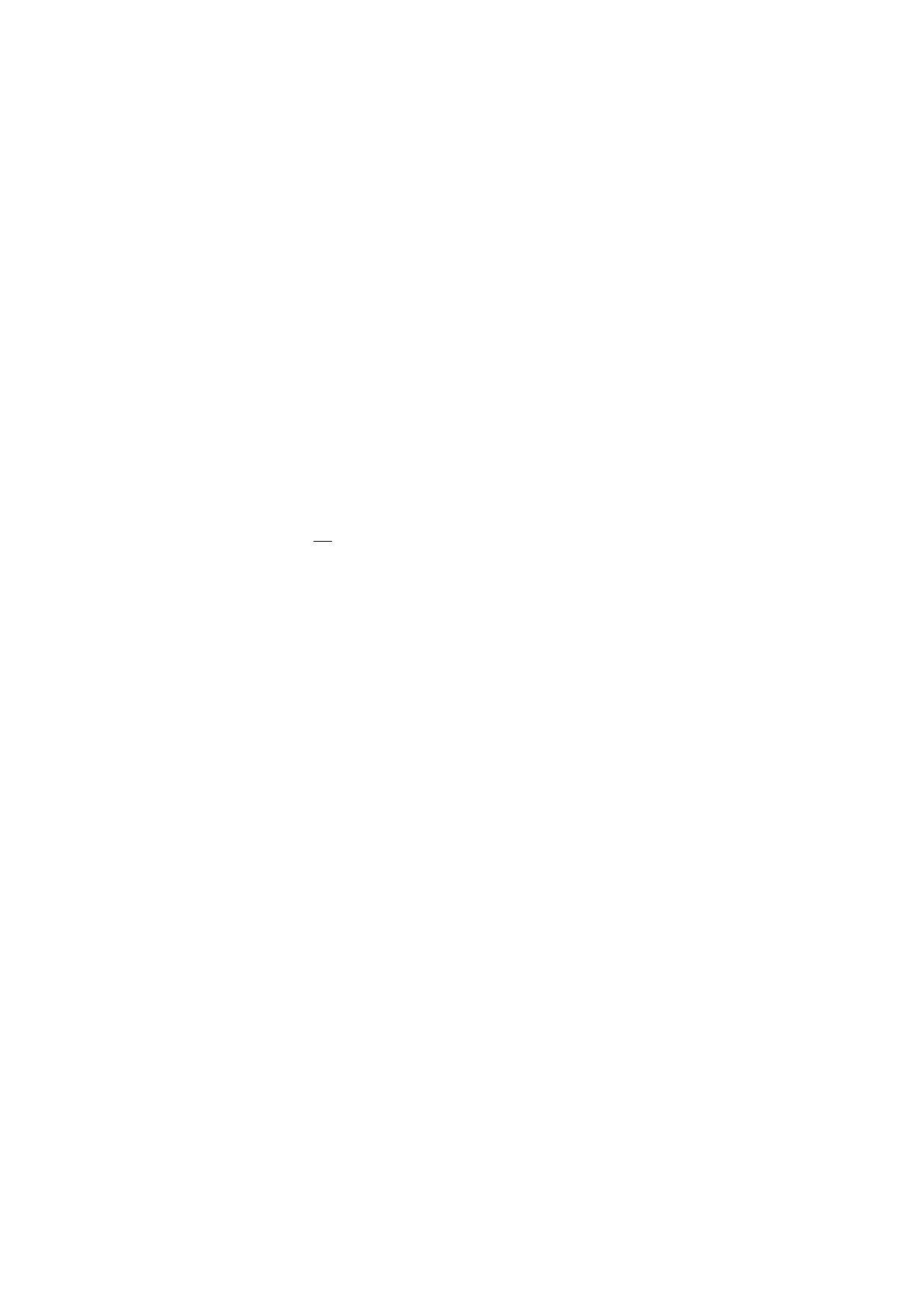
Electrochemical Two-Electrode Amperometric
Biosensor for Neurotransmitters Analysis
Moez Bouali, Student Member, IEEE, and M. Sawan, Fellow, IEEE
Polystim Neurotechnologies Laboratory, Department of Electrical Engineering
Polytechnique Montreal, Quebec, Canada
moez-2.bouali@polymtl.ca
Abstract—This paper presents a highly integrated on chip
CMOS potentiostat for neurotransmitters sensing. The circuit
maintains a constant-potential amperometry between the
reference and working electrodes. Neurotransmitter concent-
ration is converted amperometrically to an equivalent output
voltage with a slop of 0. 12 V/µA. Redox currents ranging from 1
nA to 10 µA can be measured. Analog inputs are processed and
digital outputs are generated using delta-sigma analog to digital
converter. The circuit design is well studied with taken into
account power consumption, the input dynamic range as well as
the input referred noise. The proposed architecture is
implemented with 0.18 µm 1.8 V 1-poly 6-metal CMOS process
provided by TSMC. Simulations results show that the proposed
potentiostat shows a dynamic input current range of 1 nA to 10
µA, an input referred noise of 20.3 nV/ and consumes less
than 1mA, . The proposed ADC shows an input dynamic range of
5 mV and consumes 80µW.
Index Terms—Complementary metal-oxide semiconductor
(CMOS) integrated circuits, Potentiostat, Delta-sigma modulator,
Neurochemical sensing, Microfluidic.
I. INTRODUCTION
Neurotransmitters play a key role in neural communication.
When an action potential in a pre-synaptic neuron arrives to a
synapse, neurotransmitters are released to the synaptic cleft.
The neurotransmitters diffuse to the post-synaptic neuron and
bind to its receptors. This triggers an electrical signal in the
post-synaptic neuron, thus enabling neuron-to-neuron
messaging [1]. Neurotransmitters problems such as
neurotransmitters’ dysfunctions, concentration imbalance,
composition disorder, chemical activity … may cause various
neurological disorders. Thus, monitoring the composition and
the concentration of neurotransmitters such us dopamine,
glutamine and serotonin in real-time may help researchers from
the medical field to better understand the functioning of the
human brain in order to develop new treatments to various
neurodegenerative diseases such as Alzheimer, Parkinson,
Strokes, Sclerosis, Epilepsy and Schizophrenia.
Precise and fast measurement of neuro-chemical activity is
crucial for a better understanding of neurodegenerative
diseases. Several research groups introduced various solutions
for neurotransmitters detection’s such as neurotransmitter
imaging techniques’ and electrochemical biosensors. Although
the currently available techniques for neurotransmitters
imaging such as PET and SPEC [2] techniques are fast and
considered as low-risk platform, they remain expensive
approach that utilize bulky equipments. In recent years,
Biochemical integrated sensors in particular have received a
great attention due to their small probe/electrode size, high
sensitivity, low cost, accurate and fast measurement of
chemical activity and compatibility with CMOS process. As an
alternative, Miled et al. proposed a low-voltage laboratory-on
chip for dielectrophoretic manipulation and capacitive sensing
of nano and micro particles [3]. However, it still a complex and
expensive approach which requires a complex process
fabrication since it combines different modules such as a
Microfluidic structure, a Microelectronics chip and a packaging
system which increase the consumption and the size of the
Microsystem. Zadeh et al. focused on the sensing devices and
proposed a CMOS capacitive sensor for biochemical analysis
[4]. The limitation of the previous approach is that requires a
complex calibration and remains sensitive to parasites. Janata
and Hubert focused on chemically sensitive semiconductor
devices (CSSD’s) such as the ion-selective field effect
transistor (ISFET) which operate on potentiometric mode [5].
In recent years electrochemical amperometric microsystems
have been gaining popularity in manipulating the
neurotransmitters particles [6], [7] due to their low cost, high
sensitivity and small size. Potensiostat circuit is an exemple of
amperometric chemical sensor which measures the current
arise from the oxidation or reduction at the working electrode
(WE) which is lineary proportional to the concentration Cox
(Cred) of the oxidized (reduced) species in the test solution. The
current for cathodic , and anodic processes are defined by
equation (1) and (2) respectively [8].
(1)
(2)
where z is the number of moles of electrons transferred per
mole of reactant, F is the Faraday constant = 96 487 C/mole, A
is the electrode area in square meters, Dox (Dred) is the diffusion
coefficient in m²/s for the oxidized (reduced) species, and Cox
(Cred) is the bulk concentration in moles /of the oxidized
(reduced) species in the test solution.
In this paper, the potentiostat circuit has been chosen as the
final project for the course of Smart Medical Devices. The
potentiostat system is described in Section II. Then in Section
III, the circuit level is detailed. Simulation results are presented
in section IV. Finally, Section V concludes the paper.