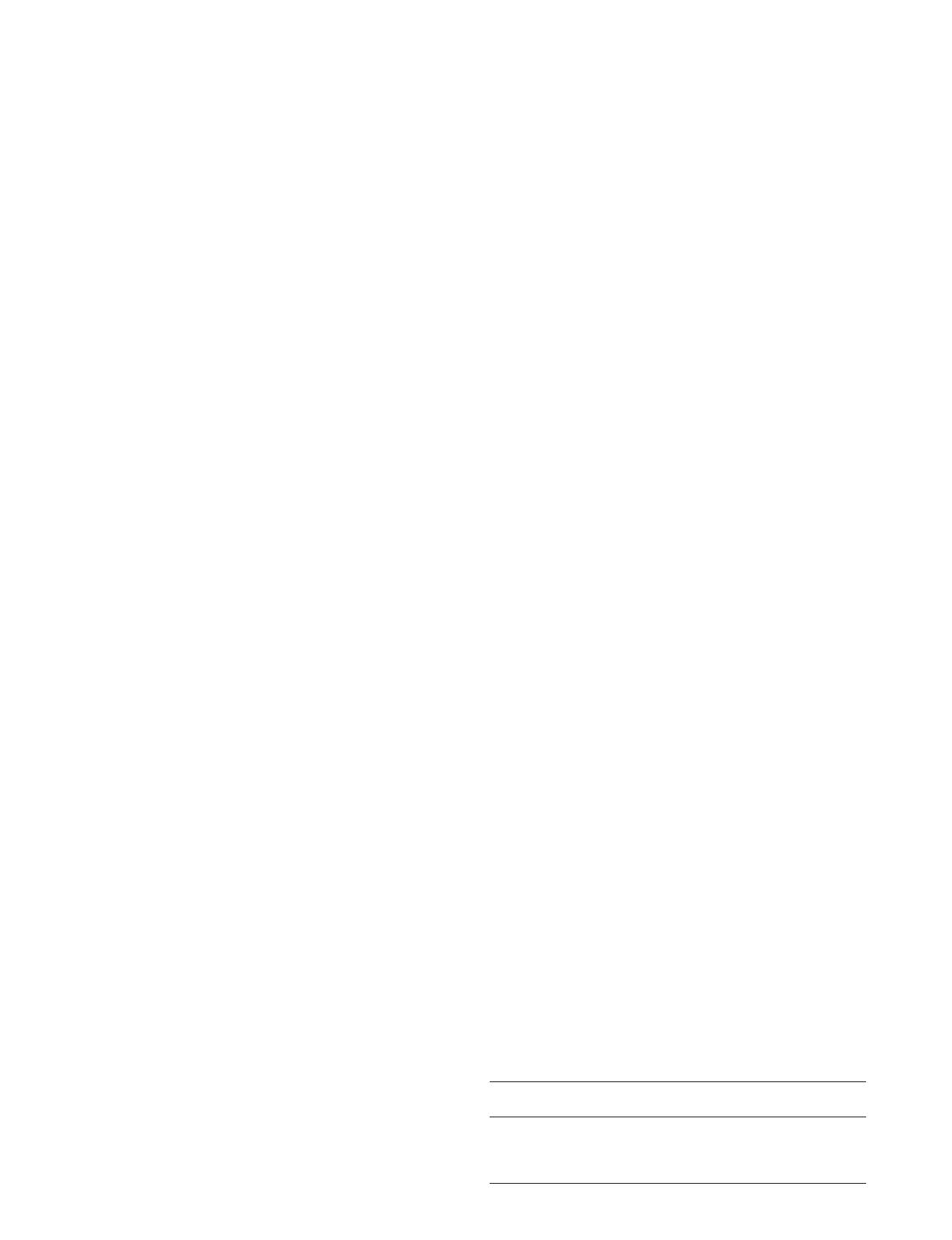
non-pathogenic bacteria. In the investigations of mag-
netic carriers for gene therapy, a viral vector which carries
the therapeutic gene is coated onto the magnetic carrier’s
surface. By holding the carrier at the target location using
external magnetic elds, the virus is kept in contact with the
tissue for a longer period of time, increasing the efciency
of gene transfection and expression (Mah et al. 2000, Mah
et al. 2002). New magnetic carriers are being developed
specially for these applications (Hughes et al. 2001), and
this is an area which shows great promise (Pankhurst et al.
2003). Viral vectors are more eective than non-viral vec-
tors for DNA delivery, but may display a signicant threat
to patients, although non-viral carriers are inherently safer
than viral carriers (Higashi et al. 2009, Kostarelos and Miller
2005, Mastrobattista et al. 2006). In contrast to the viral gene
delivery structures, the non-viral carriers are expected to be
less immunogenic, with simple preparation and a possible
adaptable surface modication (Philippi et al. 2010).
e non-viral vectors are usually made of lipids or poly-
mers with or without using other inorganic substances,
where they can also be prepared from a lipid-polymer or
lipid-polymer-inorganic hybrid (Lu 2009). Naked DNA, gen-
erally in plasmid form, is the most basic form of non-viral
transfer of a gene into a target cell (Conwell and Huang 2005,
Niidome and Huang 2002, Bigger et al. 2001, Mayrhofer et al.
2009). Non-viral delivery vectors can be classied as organic
systems such as lipid complexes and conjugated polymers,
and inorganic systems such as MNPs and gold nanoparticles
(Lee et al. 2013).
In considering the viral gene delivery vector, with its safety
concerns regarding the risk of extreme immune response
(adenovirus) and supplement mutagenesis, the usage of non-
viral vectors can overcome the safety problems mentioned
(Bharali et al. 2005). Owing to the low transferring eciency
of a naked plasmid, several chemical (liposomes) and
physical (electroporation) approaches have been exploited,
to increase their transferring eciency (Dizaj et al. 2014,
Deelman and Sharma 2009).
Targeted gene delivery in vivo
Gene delivery methods eciently present a gene of interest
in order to express its encoded protein in an appropriate host
or host cell (Kami et al. 2011). In the case of magnetofection,
the gene is attached directly to the carrier. ese carriers
commonly consist of a magnetic iron-oxide either dispersed
within a polymer matrix – such as silica, polyvinyl alcohol
(PVA), or dextran – or encapsulated within a polymer or
metallic shell (Dobson 2006, Harris et al. 2003, Neuberger
et al. 2005).
To obtain a large-sized nucleic acid molecule, the cyto-
plasm, or even the nucleus, an appropriate carrier system,
such as virosomes, cationic liposomes, and nanoparticles,
are required to deliver genes to cells, which improve cell
internalization and protect the DNA molecule from nuclease
enzymatic degradation. To achieve a suitable carrier struc-
ture, nanoparticles can be considered as good candidates for
therapeutic applications because of several reasons, as follows
(Akbarzadeh et al. 2012b): ey exist in the same size range
as proteins (Wu et al. 2008), they have large surface areas and
ability to attach to a large number of surface functional groups
(Indira and Lakshmi 2010), and they have controllable absorp-
tion and release properties, as also surface characteristics and
particle size (Dizaj et al. 2014, Nitta and Numata 2013).
Inorganic nanoparticles, polymer-based nanoparticles,
lipid-based nanoparticles and hybrid nanoparticles are four
major groups exploited in gene delivery. MNPs are inorganic
nanoparticles which are normally utilized as gene delivery
carriers. e previous study reports have demonstrated that
they are not subject to microbial attack and show also good
storage stability (Dizaj et al. 2014, Jin et al. 2014).
Magnetism-based targeted delivery was rst dened in
1978 (Widder et al. 1978). However, techniques similar to
those used for drug delivery have important potential to be
used for gene therapy. For these applications, the approach
must be adapted to account for the size and charge of nucleic
acids (Li et al. 2012).
Currently, there are three primary gene delivery meth-
ods that use viral vectors, nucleic acid electroporation, and
nucleic acid transfection. ese systems vary in eciency
(Table I). It has been demonstrated that gene delivery by
viral vectors can be highly eective, but may supplement
viral vector nucleic acid sequences into the host genome,
potentially causing undesirable eects, such as unsuitable
expression of deleterious genes (Kami et al. 2011).
e use of MNPs to increase the eectiveness of the cell-
fusion vector hemagglutinating virus Japan envelope (HVJ-E)
was represented by Morishita and others. ey found that by
associating protamine sulfate (PS)-coated MNPs to HVJ-E,
transfection was improved in vitro in BHK21 cells, even with
a reduction in the amount of HVJ-E and no proof of toxicity
(Dobson 2006, Morishita et al. 2005). However, in order for
MNPs to act as ecient carriers for DNA or pharmaceutical
drugs, the external surface of the particles must rst be mod-
ied to allow attachment of the target molecules. Molecules
can be attached to the surface of the particles in some ways,
such as employing cleavable linkers or utilizing electrostatic
interactions between the particle surface and the therapeu-
tic agent (McBain et al. 2008).
MNPs can be coated with compounds such as natural
polymers (proteins and carbohydrates) (Akbarzadeh et al.
2012c, Valizadeh et al. 2012, Akbarzadeh et al. 2013, Akbarza-
deh et al. 2012a, Akbarzadeh et al. 2012d, Akbarzadeh et al.
2012e), synthetic organic polymers (polyethylene glycol, PVA,
poly-L-lactic acid) (Akbarzadeh et al. 2013, Mollazade et al.
2013, Nejati-Koshki et al. 2013, Rezaei-Sadabady et al. 2013),
silica (Fallahzadeh et al. 2010), and gold (Kami et al. 2011,
Ebrahimnezhad et al. 2013, Pourhassan-Moghaddam et al.
2013). In the case of in vitro magnetofection, the particles are
generally coated with polyethylenimine (PEI), which attaches
Table I. Gene delivery systems (Kami et al. 2011).
Expression
type
Eciency
(%)
Cell
Viability (%) Safety
Virus *Stable, or
Transient
80–90% 80–90% Low
Electroporation Transient 50–70% 40–50% High
TF reagent ** Transient 20–30% 80–90% High
LABB_A_1014093.indd 3 9/16/2015 11:22:18 AM
S. Majidi et al.
1188