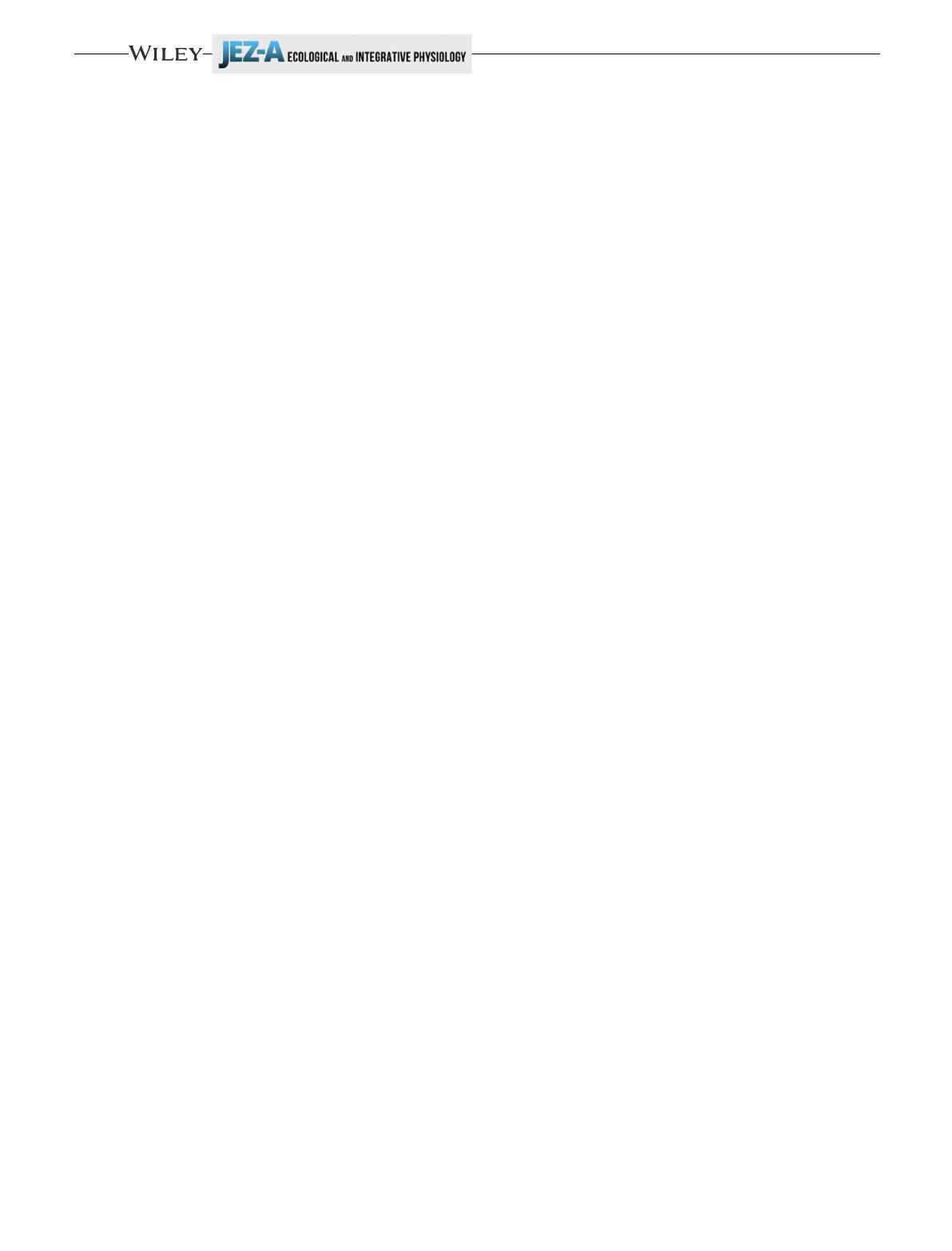
to be relatively stable and warm throughout the year (Buckley &
Huey, 2016; Pincebourde & Suppo, 2016; Sunday, Bates, &
Dulvy, 2011). In such situations, animals might not need to actively
thermoregulate to maintain suitable body temperatures, and there
should be little selection for either tight behavioral thermoregulation
or broad thermal tolerance (Buckley & Huey, 2016; Huey &
Slatkin, 1976). By contrast, nocturnal species are expected to
experience little thermal heterogeneity, but possibly cooler tem-
peratures than diurnal species (Gunderson & Stillman, 2015).
Because thermal heterogeneity is required for behavioral thermo-
regulation, this situation is expected to result in little selection for
behavioral thermoregulation, but strong selection for performance at
relatively cool temperatures. Additionally, life history stages may
vary in their capacity to thermoregulate, thermoregulatory
set‐points, or the costs associated with thermoregulation. As an ex-
treme example, developing embryos of oviparous species cannot
access thermal microenvironments outside of the egg (Cordero,
Telemeco, & Gangloff, 2018; Telemeco et al., 2016). Even free‐living
juveniles might not be able to thermoregulate as freely as adults
because of increased predation risk or other constraints. In such
situations where the capacity to thermoregulate is reduced but ex-
posure to variable thermal microenvironments is high, we expect
selection for increased thermal performance breadth (Buckley &
Huey, 2016; Gilchrist, 1995).
Crested geckos are large geckos (~35 g) endemic to tropical
forests of southeastern New Caledonia (Bauer & Sadlier, 2000). To
date, no published information is available about the physiology, life
history, or thermal ecology of crested geckos, and little information is
available about their natural history. Although first described in
the mid‐19th century, crested geckos were presumed extinct
for ~150 years before being rediscovered in 1994 (Bauer &
Sadlier, 2000; de Vosjoli, 2005). A handful of animals were exported
to the United States and Europe between 1994 and 1996, almost
entirely from their largest population on the Isle of Pines (Ile des
Pins), before exportation was banned (de Vosjoli, 2005). These cap-
tive geckos bred readily, and crested geckos are now one of the most
popular species in the commercial pet trade (de Vosjoli, 2005;
Team, 2017). A major reason for their popularity as pets is that
crested geckos thrive in human homes without additional lighting or
heating requirements, unlike most reptiles in the pet trade
(Team, 2017). This capacity suggests that crested geckos might be
broad thermal generalists, possibly because their natural habitat
(treetops in the tropics at night) offers minimal thermal hetero-
geneity. However, pet care manuals also suggest that crested geckos
perform best with temperatures between 25°C and 28°C during
activity and as low as 15°C during inactivity (de Vosjoli, 2005;de
Vosjoli & Repashy, 2014; Team, 2017). Thus, crested geckos could be
thermal specialists whose success as pets results from their thermal
requirements aligning with temperatures typically maintained within
human homes.
We tested the hypothesis that crested geckos are thermal gen-
eralists by quantifying thermoregulation and thermal performance in
the laboratory for two life‐history stages (juvenile and adult) of
captive‐reared crested gecko and compared those with air tem-
peratures in New Caledonia. We predicted that crested geckos would
not actively thermoregulate and maintain high performance across
temperatures commonly experienced. Furthermore, because juve-
niles can be harassed or even eaten by adults (de Vosjoli, 2005;
Team, 2017), potentially forcing them to inhabit marginal habitats,
we predicted that juveniles would have broader thermal performance
breadth than adults. Finally, we directly compared thermal behavior
and performance to illuminate how natural selection has shaped
thermal set‐points in this species. Based on this comparison, we
propose that natural selection shapes thermoregulatory set‐points to
approximate the lower inflection point on the TPC (lower T
d
) because
performance benefits should most outweigh the costs of metabolic
demand and overheating risk at this temperature.
2|METHODS
2.1 |Animal acquisition and maintenance
We obtained adult crested geckos from commercial breeders in
Fresno, CA in March 2018 and transported them to the laboratory at
California State University, Fresno. All animals were ~1 year of age at
acquisition and the result of captive breeding, likely for many gen-
erations. Before experiments were carried out, adult geckos (N= 18;
females = 9, males = 9) were housed in captivity for ~11 months and
were ~2 years old at the start of the trials. We performed trials with
N= 17 juveniles produced from the eggs of these adults, and these
juveniles ranged from 7.2 to 9.4 months of age at the start of the
trials. One juvenile died before its final trial, and data are only in-
cluded in the analyses for which it was alive.
Before conducting the experiments, animals were housed in a
climate‐controlled room with temperature maintained at ~25–27°C,
relative humidity of ~60%, and a 12:12 inverted light cycle (lights on
from 18:00 to 6:00). Lizards were housed in glass enclosures with
wire mesh tops (45.72 × 45.72 × 60.96 cm, L×W×H) in groups of
either one male and two females, or one male and one female. Adult
lizards were uniquely marked with visual implant elastomer (VIE)
tags (Northwest Marine Technology Inc., Shaw Island, WA) injected
under the skin of the limbs for permanent identification. Enclosures
were outfitted with artificial greenery and egg carton for climbing
and hiding, and a mixture of moist peat moss and vermiculite for
oviposition. We obtained eggs from six females and incubated them
in vermiculite with a water potential of −150 kPa (Telemeco, 2014)at
room temperature (~20°C). Hatchlings were not marked with VIE but
were instead housed individually for identification, initially within
16‐oz plastic cups with mesh lids and then in 32‐oz plastic cups with
mesh lids after 2 months. An egg crate was placed within these cups
to provide climbing structures and hides. All enclosures were misted
with water twice daily to ensure ad libitum water availability and
high humidity within enclosures. We provided all geckos a fresh dish
of crested gecko diet (Breeding Formula for Reproductive Adult
Geckos; Pangea Reptile LLC; mixed by following manufacturer's
88
|
APARICIO RAMIREZ ET AL.
24715646, 2021, 1, Downloaded from https://onlinelibrary.wiley.com/doi/10.1002/jez.2388 by Univ Of California Santa Cruz - UCSC, Wiley Online Library on [14/11/2024]. See the Terms and Conditions (https://onlinelibrary.wiley.com/terms-and-conditions) on Wiley Online Library for rules of use; OA articles are governed by the applicable Creative Commons License