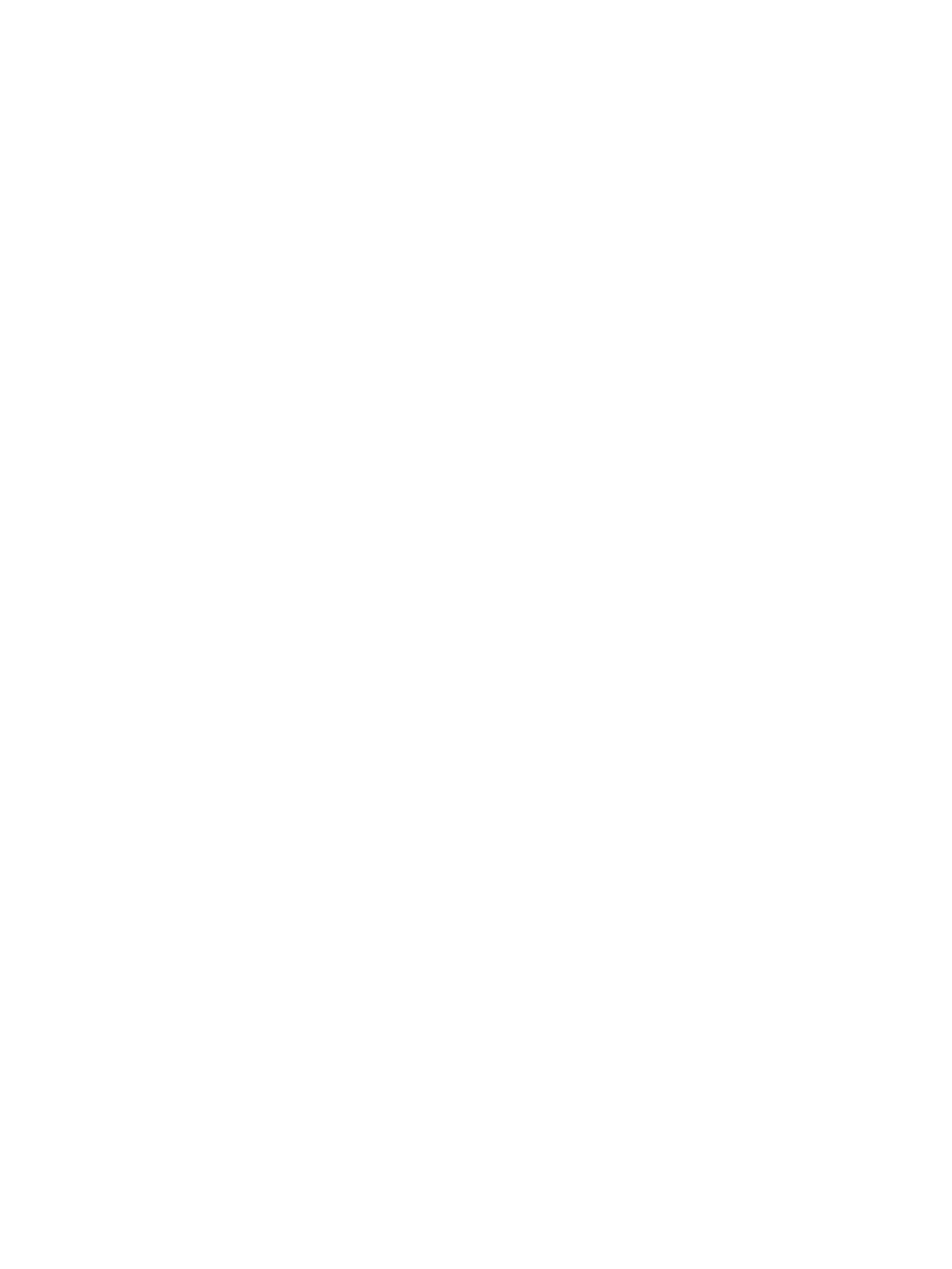
Journal of Water Process Engineering 58 (2024) 104838
2
1. Introduction
The pharmaceutical industry has rapidly grown over the past two
decades, becoming a global economic powerhouse. Recent research re-
veals that global antibiotic consumption reached 40.1 billion dened
daily doses in 2018. In the pharmaceutical industry, water serves mul-
tiple purposes, such as raw materials, components, and solvents in
formulating, producing, and processing pharmaceutical products [1].
About 23 % of the water supply is estimated to go towards its operation,
which results in the generation of highly toxic and contaminated
wastewater that demands costly, multistage treatment to meet regula-
tory requirements [2]. Pharmaceutical wastewater has a high concen-
tration of ammonia‑nitrogen, chroma, organic material, and nutrients. It
exhibits elevated levels of chemical oxygen demand (COD), biological
oxygen demand (BOD), and chemical oxygen concentration (COC).
Reports indicate that nearly 200 pharmaceutically active compounds
(PhACs) have been found in various water sources, from wastewater to
surface water to groundwater [3]. Conventional wastewater treatment
plants (WWTPs) are not designed to degrade emerging pollutants like
PhACs. Studies have found high concentrations of antibiotics, such as
cephalexin (CEX), azithromycin (AZM), and ciprooxacin (CIP), in
treatment plant efuents [4]. Several methods, such as activated sludge
[5], anaerobic treatment [6], constructed wetlands [7], adsorption [8],
coagulation/occulation [9], membrane [10], and ozone/hydrogen
peroxide treatment integrated technology [11], were explored for
pharmaceutical wastewater treatment. However, conventional waste-
water treatment processes are not designed to degrade emerging phar-
maceutical pollutants.
Recently, membrane technology has become increasingly popular for
large-scale water treatment systems, especially in developed countries
[12]. Basically, a membrane is a semi-permeable barrier, allowing some
substances from the source uids to pass through while selectively
preventing others. Contaminant separation is dependent on attributes
like charge and size [13]. However, membrane clogging and fouling due
to the deposition of solutes over the membrane surface or gel layer
formation by concentration polarization near pores have resulted in
permeability loss, which depends upon the module type (crossow or
dead-end), membrane materials, applied pressure, and crossow rate
[14]. Such intervention is time-consuming, chemical-intensive, labor-
intensive, and possibly destructive to membranes, all of which have a
bigger impact on costs. Catalytic membranes (CMs) can be applied to
drive a continuous treatment process, generating reclaimable treated
water. Catalytic properties can be integrated onto and throughout the
surface of the membrane, introducing an advanced technology for
wastewater treatment referred to as membrane catalytic reactors
(MCRs). CMs have a dual role, as they anchor the catalyst on their
surface or embed it within their structure. This dual function combines
ltration and catalysis capabilities, allowing simultaneous pollutant
oxidation to maintain membrane cleanliness and delay membrane
fouling, enabling easy catalyst reapplication and enhancing the process
intensication approach [15,16]. It integrates membrane separation
with catalytic reactions within a singular unit, resulting in increased
conversion rates, enhanced selectivity, and a more streamlined, cost-
efcient reactor design that can serve the gaps in conventional treat-
ment processes [17].
Lu et al. synthesized a catalytic nanobrous membrane known as
Co
x
O
y
@carbon catalytic nanobrous membrane (Co
x
O
y
@CCNM) by
facilitating the in-situ growth and subsequent pyrolysis of ZIF-67 on the
nanobers [18]. This membrane enables rapid, durable, and continuous
degradation of antibiotic compounds such as tetracycline (TC), CIP, and
sulfamethoxazole (SMZ) through a gravity-driven ow-through ltra-
tion process. The novel ZIF-67-derivative-functionalized Ti-based CMs
(ZTM-T) demonstrated exceptional efciency in removing SMX, reach-
ing an impressive removal rate of 96.3 %. This level of removal was
signicantly higher, 216.7 times greater than membrane ltration alone
and 3.8 times higher than peroxymonosulfate (PMS) treatment alone. In
addition, a potential mechanism for the degradation of SMX was
investigated using a combination of density functional theory analysis
and high-performance liquid chromatography-mass spectrometry [19].
MCRs may contain conductive or chemically active components, i.e.,
external stimuli such as light, electrical current, chemical activation,
enzymatic response, or any combination of these, which can boost the
concentration and destruction of the contaminants [20].
Here, several emerging technologies, starting from membrane-based
separation and membrane bioreactors, various AOPs, and advanced
MCRs, are critically evaluated for continuous treatment to enhance the
scale-up condence from lab-to-pilot-to-industry. A paradigm shift is
necessary to tackle the quick-changing scenario on real eld scenarios
for treating complex pharmaceutical wastewater by developing novel
MCR-based technology. Membrane-based emerging technologies help to
transform the existing wastewater treatment plant technologically faster
and more efciently for the removal of PhACs. This holistic approach
addresses the long-term sustainability of urban water systems, with
“blue” representing freshwater supplies, “green” representing environ-
mentally friendly technologies, and “grey” representing wastewater
purication. These systems can enhance water quality and protect
ecosystems by eliminating organic contaminants, heavy metals, and
pharmaceuticals from wastewater through the combined power of
photocatalysis and membrane separation.
The current review manuscript has conducted an extensive literature
survey on essential aspects, such as composition variation in pharma-
ceutical wastewater and innovation in treatment processes, especially in
advanced oxidation processes (AOPs). The novel content in the present
review paper compared to the existing literature on pharmaceutical
wastewater treatment over the last ve years is mentioned in Table 1.
Most of the review manuscripts focused on general technological ad-
vancements [21,22] or the hybrid treatment process that includes con-
ventional and emerging technologies, such as adsorption followed by
electrochemical oxidation [23], combined biological, membrane, and
ultrasonication [24], AOPs integrated with nanocatalyst [25], AOP with
immobilized photocatalysts on carbon nanotubes [26], and integration
of photocatalysis and membrane separation technology [27]. The
membrane separation technology is considered a promising method for
the separation of solid from liquid [28,29], which can be utilized as a
suitable carrier for novel and effective nanoscale photocatalytic material
for the simultaneous degradation of PhACs and permeation of treated
water in a continuous way.
2. Pharmaceutical wastewater: an overview
Water, a vital component in pharmaceutical production, is a raw
material in producing different pharmaceutical products, active phar-
maceutical ingredients, formulations, analytical reagents, and
compendia articles. This necessitates a reliable supply of clean water for
the industry [1,36]. The pharmaceutical industry is extremely water-
dependent, resulting in numerous wastewater-generating processes.
The sheer volume and composition of wastewater in the pharmaceutical
industry vary by operation and production rate, with no standard
wastewater composition due to the heterogeneity of drugs used. The
efuent is highly toxic and dangerous to the biota and has an intense
hue. Non-biodegradable organic matter such as antibiotics, animal and
plant steroids, analgesics, beta-lactamides, reproductive hormones, and
metals, such as chromium, cadmium, mercury, lead, and nickel, are
found in large quantities in pharmaceutical wastewater [37]. The Cen-
tral Pollution Control Board of India regulates the release of such haz-
ardous waste into the environment. Efuent discharge restrictions for
the selected countries are shown in Table 2. Multiple studies have used
pharmaceutical wastewater to classify pharmaceutical wastewater by
using pH measures, total dissolved solids (TDS), BOD, COD, biode-
gradability, alkalinity, and ammonia‑nitrogen concentration. While
characterizing pharmaceutical wastewater in India, Damodhar and
Reddy found that the River Uppanar had TDS levels ranging from 354.38
R. Kumar et al.