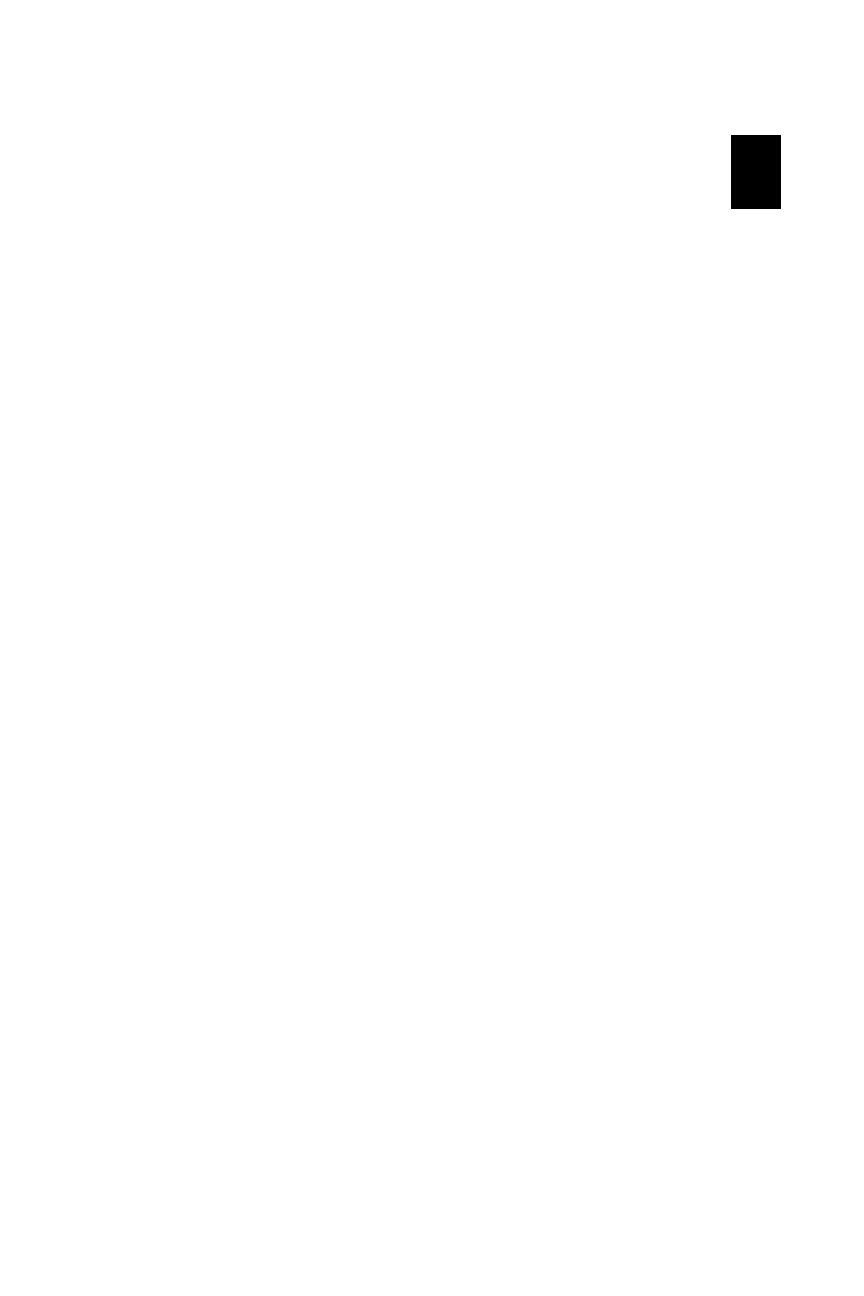
CO
2
corrosion of mild steel 7
Aria Kahyarian
1
, Mohsen Achour
2
and Srdjan Nesic
1
1
Ohio University, Athens, OH, United States;
2
ConocoPhillips, Bartlesville, OK, United States
7.1 Introduction
Produced oil and gas are always accompanied by some water and varying amounts of
carbon dioxide and in some cases hydrogen sulfide and organic acids. All of these may
affect the integrity of mild steel. This has been known for over 100 years, yet internal
corrosion of pipelines and other facilities made from mild steel still represents a chal-
lenge for the oil and gas industry. Although many corrosion-resistant alloys are able to
withstand this type of corrosion, it is a matter of economics: mild steel is still the most
cost-effective construction material. The price of failure due to internal corrosion is
enormous, both in terms of direct costs, such as repair/replacement costs and lost pro-
duction, and indirect costs, such as environmental cost and impact on the downstream
industries.
The following text summarizes the current degree of understanding of CO
2
corro-
sion of mild steel exposed to aqueous environments. Much has been understood about
the basic mechanisms, enabling construction of mechanistic prediction models, but
many challenges remain, particularly when it comes to effects of corrosion product
layers, multiphase flow, additional species, hydrocarbon composition, inhibition,
etc. The following sections start out with describing the basic physicochemical phe-
nomena underlying CO
2
corrosion and gradually move to more complicated situations,
culminating with the list of multifaceted real-life challenges seen in the field.
7.2 Water chemistry in CO
2
corrosion
Carbon dioxide (CO
2
) is a stable, inert, and noncorrosive gas. However, upon disso-
lution in water and a subsequent hydration reaction, a more reactive chemical species,
carbonic acid (H
2
CO
3
), is formed. This reaction is followed by dissociation reactions
to form bicarbonate HCO3ion, carbonate CO32ion, and hydrogen (H
þ
) ion,
resulting in an acidic and corrosive solution. The reactions associated with these chem-
ical equilibria and their corresponding mathematical relationship are listed in
Table 7.1.
The chemical equilibria and water chemistry associated with dissolved CO
2
and its
carbonate derivatives have been extensively studied [1e9]. The first step, CO
2
disso-
lution, is described via Eq. (7.i), where KCO2is the proportionality constant and can be
expressed as Henry’s constant for the simplest case of ideal gas and ideal solution. The
nonideal behavior of the gas phase, represented by fugacity coefficient, and the liquid
Trends in Oil and Gas Corrosion Research and Technologies. http://dx.doi.org/10.1016/B978-0-08-101105-8.00007-3
Copyright ©2017 Elsevier Ltd. All rights reserved.