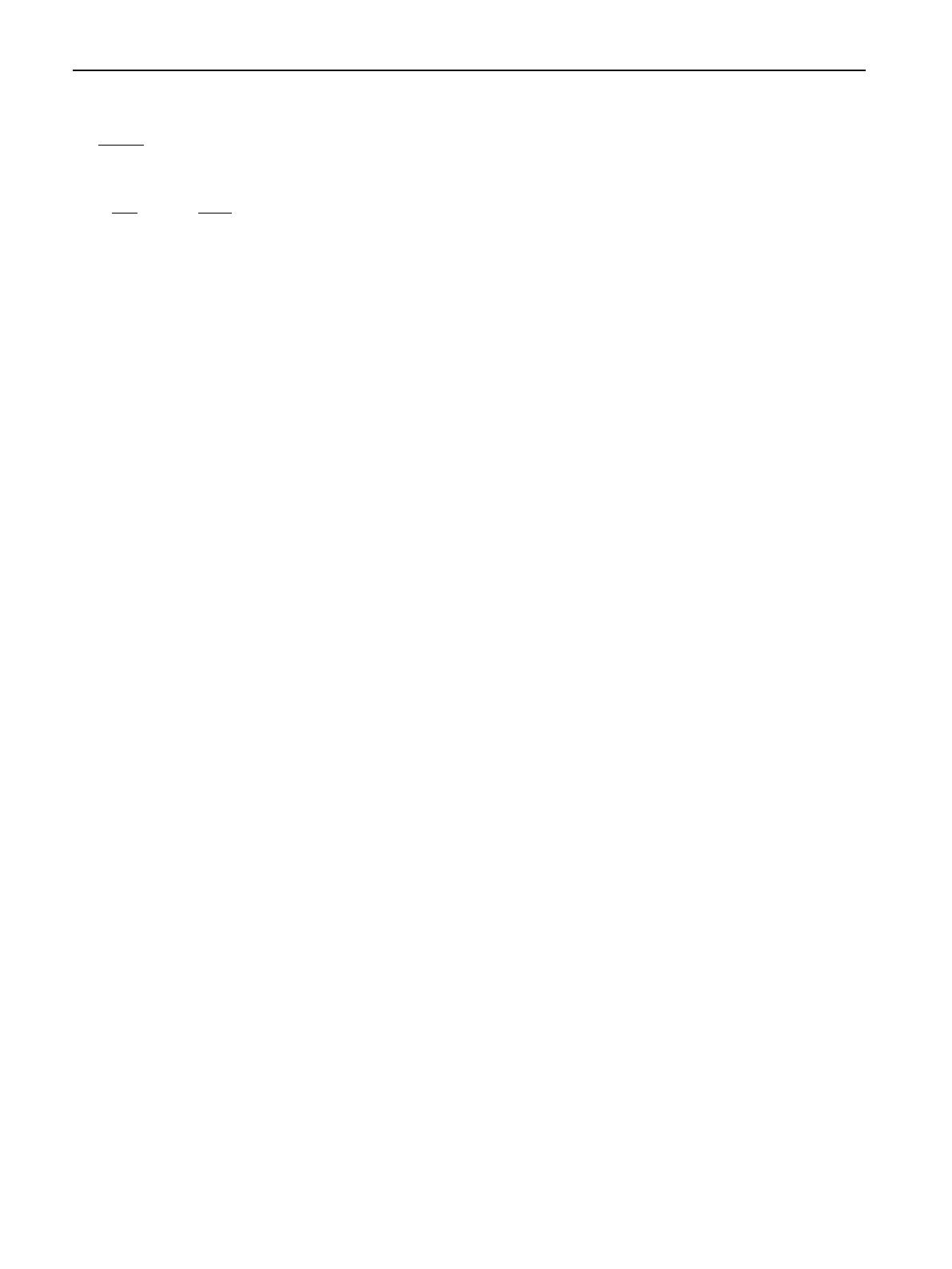
ing that this compound adsorbs according to Langmuir
adsorption isotherm where,
h¼KC
KC þ1:ð10Þ
With
K¼1
55:5
exp DGads
RT
;ð11Þ
where Kis the equilibrium constant for the adsorption process,
Cis the concentration of the inhibitor.
The value of equilibrium adsorption constant obtained
from this isotherm is about 4.21 ·10
4
L mol
1
suggesting a
chemically adsorbed film (Villamil et al., 2002).
It is well known that values of DG
ads
of the order of
20 kJ mol
1
or lower indicate a physisorption mechanism;
those of the order of 40 kJ mol
1
or higher involve charge
sharing or a transfer from the inhibitor molecules to the metal
surface to form a co-ordinate type of bond (Donahue and
Nobe, 1965; Kamis et al., 1991).
The largest negative value of DG
ads
(36.94 kJ mol
1
) ob-
tained here indicates that this inhibitor is strongly adsorbed
onto the copper surface and is typical to chemisorption.
4. Conclusions
The main conclusions drawn from this study are:
– MMI inhibits the corrosion of copper in 0.5 M H
2
SO
4
.
– The inhibition is due to adsorption of the inhibitor mole-
cules on the copper surface.
– The adsorption of MMI on the copper obeys the Langmuir
adsorption isotherm model.
– Results obtained from dc polarization, ac impedance and
weight loss techniques are in reasonably good agreement
and show increased inhibitor efficiency with increasing
inhibitor concentration.
References
Abdel-Gaber, A.M., Abd-El-Nabey, B.A., Sidahmed, I.M.,
El-Zayady, A.M., Saadawy, M., 2006. Corros. Sci. 48, 2765.
Bouyanzer, A., Hammouti, B., Majidi, L., 2006. Mater. Lett. 60, 2840.
Chadwick, D., Hashemi, T., 1979. Surf. Sci. 89, 649.
Chauhan, L.R., Gunasekaran, G., 2007. Corros. Sci. 49, 1143.
Curkovic, H.O., Stupnis
ˇek-Lisac, E., Takenouti, H., 2009. Corros. Sci.
51, 2342.
da Costa, S.F.L.A., Agostinlo, S.M.L., Rubim, J.C., 1990. J. Electro-
anal. Chem. 295, 203.
Donahue, F.M., Nobe, K., 1965. J. Electrochem. Soc. 112, 886.
Fallavena, T., Antonow, M., Gonc¸ alves, R.S., 2006. Appl. Surf. Sci.
253, 566.
Gas
ˇparac, R., Stupnis
ˇek-Lisac, E., 1999. Corrosion 55, 1031.
Gas
ˇparac, R., Martin, C.R., Stupnis
ˇek-Lisac, E., 2000a. J. Electro-
chem. Soc. 147, 548.
Gas
ˇparac, R.R., Martin, C.R., Stupnis
ˇek-Lisac, E., Mandic, Z., 2000b.
J. Electrochem. Soc. 147, 991.
Hukovic-Metikos, M., Babic, R., Grutac, Z., 2002. J. Appl. Electro-
chem. 32, 35.
Jang, J., Ishida, H., 1992. Corros. Sci. 33, 1053.
Kamis, E., Belluci, F., Latanision, R.M., El-Ashry, E.S.H., 1991.
Corrosion 47, 677.
Larabi, L., Benali, O., Mekelleche, S.M., Harek, Y., 2006. Appl. Surf.
Sci. 253, 1371.
Ling, Y., Guan, Y., Han, K.N., 1995. Corros. Sci. 51, 367.
Ma, H., Chen, S., Yin, B., Zhao, S., Liu, X., 2003. Corros. Sci. 45, 867.
Mansfeld, F., 1981. Corrosion 37, 301.
Mattson, E., Bockris, J.O’M., 1586. Trans. Faraday Soc. 55, 1586.
McCafferty, E., 1997. Corros. Sci. 39, 243.
Modestov, A.D., Zhou, G., Schwinsberg, D.P., 1994. Corros. Sci. 36,
1931.
Moretti, G., Guidi, F., 2004. Corros. Sci. 46, 387.
Moretti, G., Guidi, F., Grion, G., 2004. Corros. Sci. 46, 387.
Rahim, A.A., Rocca, E., Steinmetz, J., Kassim, M.J., 2008. Corros.
Sci. 50, 1546.
Raja, P.B., Sethuraman, M.G., 2008. Mater. Lett. 62, 113.
Saban, A., Kalman, E., Telegedi, J., 1998. Electrochim. Acta 43, 159.
Smyrl, W.H., 1981. Copper dissolution kinetics in H
2
SO
4
. In: Bockris,
O’M., Conway, B.E., Yeager, E., White, R.E. (Eds.). Comprehen-
sive Treatise of Electrochemistry, vol. 4. Plenum Press, New York,
p. 116.
Stupnis
ˇek-Lisac, E., Galic, N., Gas
ˇparac, R., 2000. Corrosion 56,
1105.
Stupnis
ˇek-Lisac, E., Loncaric Bozic, A., Cafuk, I., 1998. Corrosion 54,
713.
Stupnis
ˇek-Lisac, E., Gazivoda, A., Madzarac, M., 2002. Electrochim.
Acta 47, 4189.
Tommesani, L., Brunoro, G., Frignani, A., Monticelli, C., Dal Colle,
M., 1997. Corros. Sci. 39, 1221.
Villamil, R.F.V., Corio, P., Rubin, J.C., Agostinho, S.M.L., 2002. J.
Electroanal. Chem. 535, 75.
Villamil, R.F.V., Cordeiro, G.G.O., Matos, J., D’Elia, E., Agostinho,
S.M.L., 2002. Mater. Chem. Phys. 78, 448.
Wu, J.S., Nobe, K., 1981. Corrosion 37, 223.
Wu, X., Ma, H., Chen, S., Xu, Z., Sui, A., 1999. J. Electrochem. Soc.
146, 1847.
Yoshida, S., Ishida, H., 1985. Appl. Surf. Sci. 20, 497.
Yoshida, S., Ishida, H., 1995. Appl. Surf. Sci. 89, 39.
Zvauya, R., Dawson, J.L., 1994. J. Appl. Electrochem. 24, 943.
Inhibiting effects of 2-mercapto-1-methylimidazole on copper corrosion in 0.5 M sulfuric acid 235