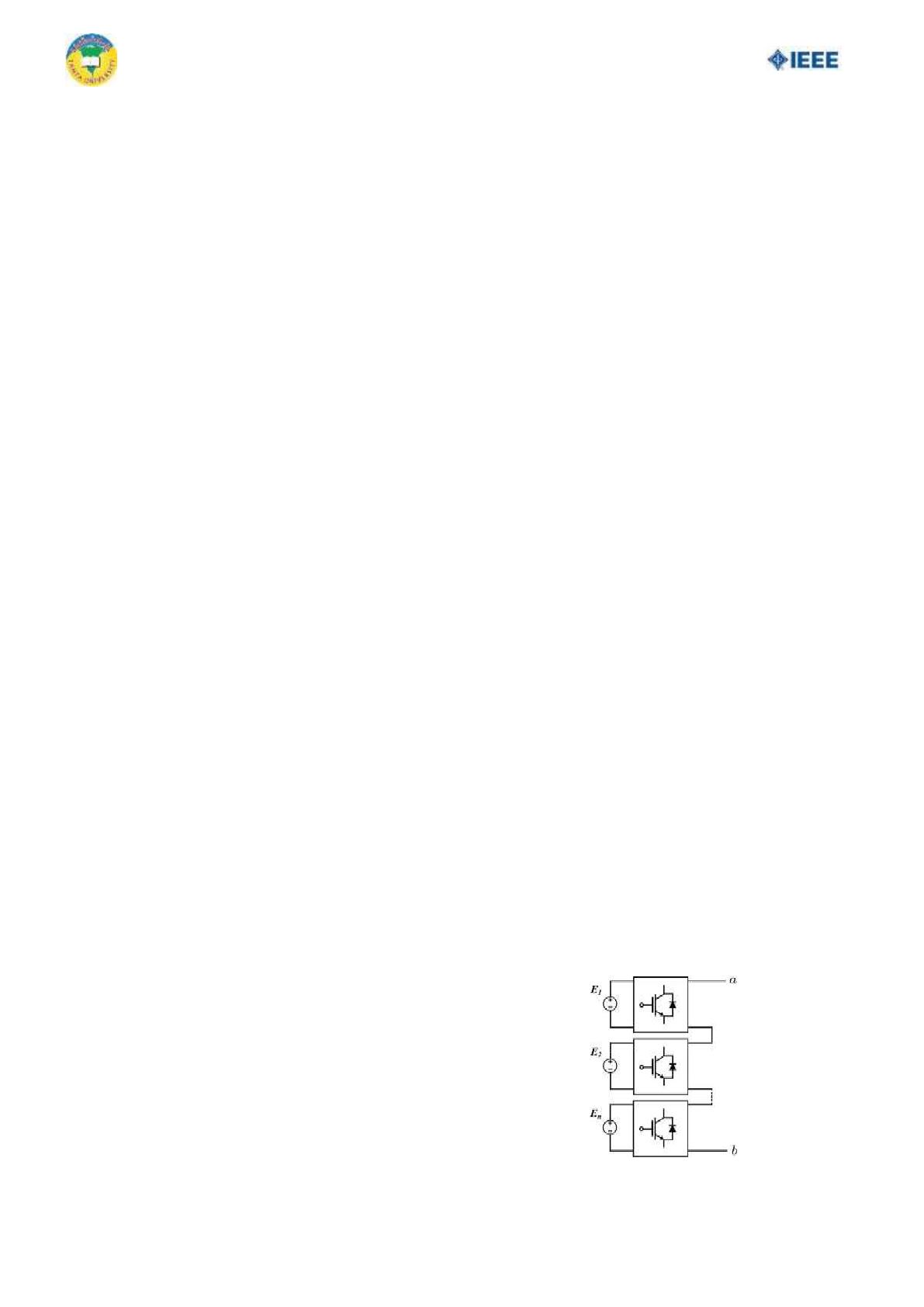
Authorized licensed use limited to: University of Gothenburg. Downloaded on July 25,2020 at 19:22:13 UTC from IEEE Xplore. Restrictions apply.
2019 21st International Middle East Power Systems Conference (MEPCON), Tanta University, Egypt
Cascaded Multilevel Split-Source Inverters:
Analysis and Modulation
Mahmoud AbdulSalam, Sherif M. Dabour, Essam M. Rashad
Power Electronics and Electric Drives (PEED) Research Lab
Faculty of Engineering, Tanta University, Tanta, Egypt
Abstract—A new cascaded multilevel inverter topology is
proposed in this paper. This topology extends the idea of the
recently introduced Split-Source Inverter (SSI) to the cascaded
Multi-Level Inverter (MLI) configurations. This structure
provides the boosting capability in conjunction with the dc to ac
conversion in a single unit with reduced passive elements.
Therefore, it represents a good alternative of the well-known Z-
Source MLIs. Two different configurations of the proposed
inverter are introduced based on the configuration of each SSI
unit. The topological structure, operating principle and
modulation techniques for each configuration of the proposed
inverter are presented. The validity of the analysis and the
performance of the presented topology are investigated via
simulation study.
Keywords—Cascaded multilevel inverter, Split-source
inverter, phase-shift carrier modulation, level-shifted carrier
modulation
I.
INTRODUCTION
RID-CONNECTED PV farms have different power
converter connections and configurations, centralized,
string, and microinverters [1]. Centralized converters market
share is lowered by time due to high voltage connections,
shading, partial shading, and power devices rating, cost, and
size problems. String and microinverters shipment are
increased a year by year and expected to reach 9GW by 2021.
Microinverters succeeded in the market as modularity, and
scalability of the systems is achieved, this leads to easy, and
fast maintenance in zero outage time. As the price of PV
panels cost is lowered, power converter manufacturers also
need to enhance efficiency, reduce the size, and lowering the
cost. Performance enhancement of power converter can be
achieved by using Sic or Gan devices, but it’s still not
preferred as a cost-wise [2].
Multilevel inverters (MLI) are the standard solution of the
PV applications if low devices’ ratings are needed [3].
Different technologies are available to use for grid-connected
systems [4]. Among the multilevel inverters, the cascaded H-
Bridge topologies has the advantages of modularity. Because
it can be constructed from multiple units of single-phase H-
bridge modules as shown in Fig. 1. To achieve a module-level
monitoring, and control such as MPPT, each PV panel is
connected to microinverter that is connected in series with
each other to get required system voltage. Microinverter
consists of the dc-dc stage called power optimizer (MPPT)
and dc-ac stage. In this case, each module of the cascaded MLI
is constructed as shown in Fig. 2(a) from front-end boost
converter fed H-bridge inverter. On the other hand, each unit
could be a single-stage power converter (SSC) such as in Fig.
2(b), which uses a single-phase impedance source inverters
(ZSI). The SSC reduces the system cost, size, and enhances
the performance, and efficiency. The ZSI utilizes four passive
elements in the impedance network and suffers from [5]
discontinues input current, besides
using additional switching states.
Utilizes four passive elements
A modified ZSI that overcomes discontinuity of input
current called quasi-ZSI (QZSI) suffers from pulsating
inverter bridge dc voltage that arises switches voltage stresses
[6]. Another SSC suggested technology is the split-source
inverter [7]. The SSI consists of a single inductor and a diode
for each inverter leg. It has two different topologies termed in
this paper by P-type and N-type as shown in Fig. 2(c) and (d).
The SSI offers many advantages compared to other SSC [8]
Same number of switches
The lowest number of passive elements
Continuous supply current and lowered bridge
capacitor voltage stress
Use the same switching states and modulation
techniques of the VSI
No special added switching states
The SSI has been used as a single-stage two-level inverter
and can be extended to multi-level inverters [9], [10]. Split-
source H-bridge (SSHB) is a perfect submodule configuration
to be a power conditioning stage between PV panels and load,
or grid terminals. Cascaded H-bridge can be built using the
aforementioned microinverter as it achieves module-level
control.
This paper proposes a new cascaded multilevel inverter
topology based on split-source H-bridge submodules. The
proposed topology improves the performance of the
conventional single-stage and multilevel boosting topologies
in terms of reducing the number of passive elements in each
H-bridge unit. The operating principles and modulation
techniques of the proposed inverter are introduced in this
paper. Finally, a seven-level topology is selected as a case
study to verify the presented analysis via simulation results
using Matlab/Simulink.
Fig. 1. Schematic diagram of the conventional CHB inverter.
978-1-7281-5289-9/19/$31.00 ©2019 IEEE