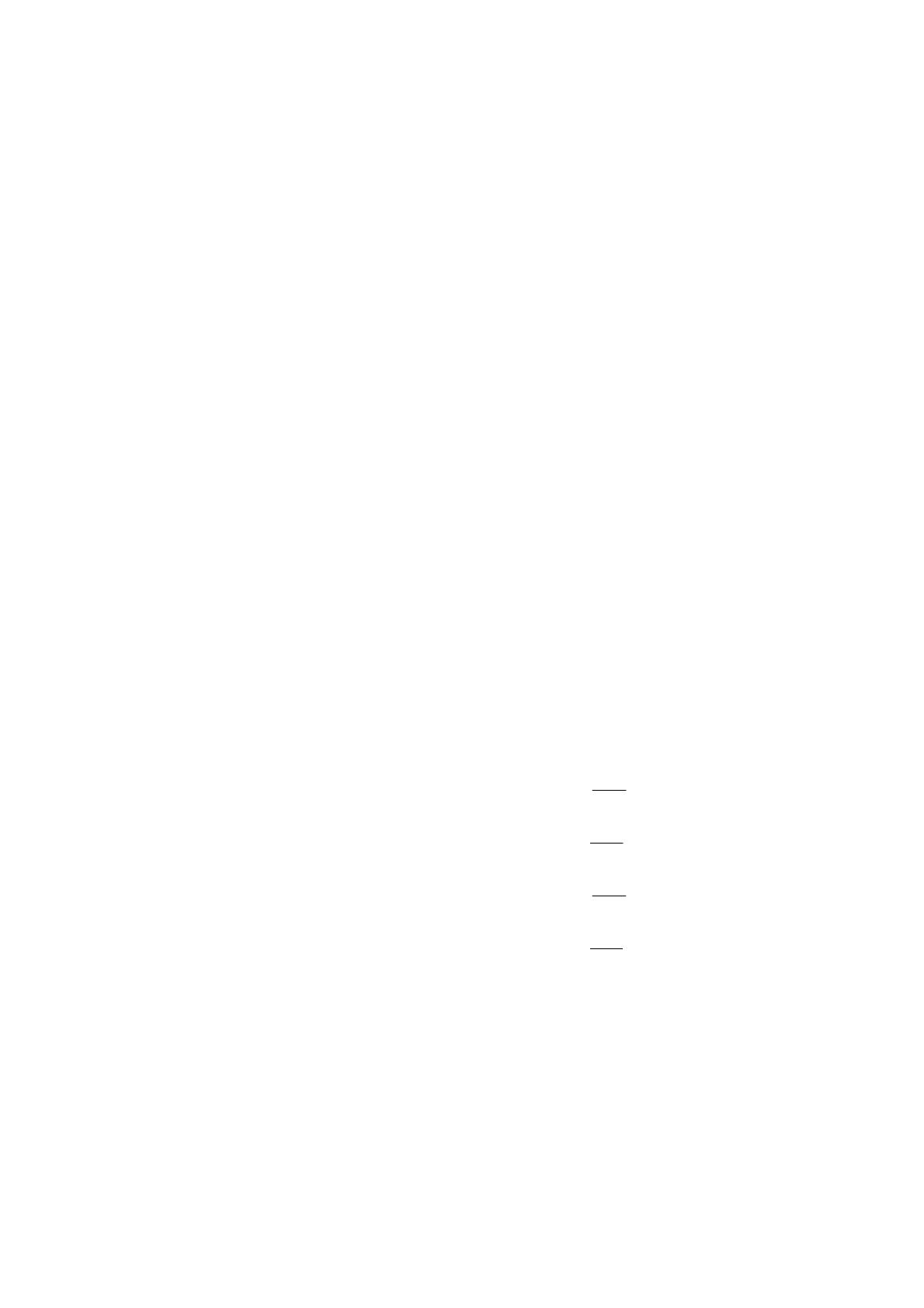
Use of an LC filter to improve the quality of energy in a DFIG joined to the grid
M. Hacil, A. L. Nemmour, A. Khezzar and M. Boucherma
Département d’Electrotechnique, Université Mentouri, Constantine, Algérie. hacil2002@yahoo.fr
Phone/Fax: +0021331819013
Abstract- In this paper, the application of an LC filter to
medium voltage rotor DFIG fed by inverters with switching
frequencies below fc is described. It is shown that the use of
LC filter gives several advantages for the drive system design,
how ever; a good system understanding is needed to utilize
these advantages. A vector control strategy for a doubly-fed
induction generator (DFIG) driven by a wind turbine and
connected to grid utility is presented. Although the rotor
inverter is controlled with an optimized space vector
modulation algorithm (SVM), the rotor currents are not
perfectly sinusoidal which causes undesirable fluctuations of
generating active and reactive stator powers. In order to more
improve the rotor current waveform, a second-order output
LC filter is inserted between the inverter and the rotor circuit.
Simulation studies of the proposed power generation system
were carried out. The obtained results show that the control
performances of the DFIG are clearly improved with the
proposed solution.
I. INTRODUCTION
Wind energy is one of the most important and promising
sources of renewable energy all over the world, mainly
because it is considered to be nonpolluting and
economically viable. At the same time, there has been a
rapid development of related wind turbine technology.
Based on the rotor construction, induction generators can be
divided into wound-rotor induction generators and squirrel-
cage induction generators. The stator of wound-rotor
induction generators is directly connected to the grid utility,
and the rotor is also connected to the utility grid through a
power converter set. Since both the stator and rotor of
wound-rotor induction generators can transfer power, they
are also termed as double-fed induction generators. The
doubly fed induction generator (DFIG) can supply power at
constant voltage and constant frequency while the rotor speed
varies. This makes it suitable for variable speed wind energy
applications. Additionally, when a bidirectional AC-AC converter
is used in the rotor circuit, the speed range can be extended above
synchronous speed and power can be generated both from the
stator and the rotor. An advantage of this type of DFIG drive is
that the rotor converter need only be rated for a fraction of the
total output power, the fraction depending on the allowable sub-
and super-synchronous speed range.
[1][2].
High-frequency voltage-source inverters (VSI) have
been widely used to synthesize sinusoidal voltages for
many applications, such as uninterruptible power suppliers
(UPS), AC power sources and reactive static compensators.
As these inverters present a high total harmonic distortion
(THD) in the output voltages owing to the high-frequency
harmonics components introduced by the modulation, it is
often necessary to equip these converters with low-pass LC
filters.
These kind of filters have been designed to obtain the
highest natural frequency of the filter that meets a specified
maximum acceptable THD in the output voltages, taking
into consideration the modulation strategy used, it is
possible to make LC filter and command the inverter with
PWM or SVM for eliminate the high frequency in the
output signal.
This paper proposes a novel circuit configuration for
eliminate a high commutation frequency of the doubly fed
induction generator. This scheme employs a parallel LC
power filter connected to the rotor converter output. A
detailed mathematical model of the resulted system is
given.
II. GLOBAL SYSTEM MODELING
a- Vector control of the DFIG:
The DFIG must supply constant voltage and frequency at the
stator terminals irrespective of the shaft speed. Unlike the grid-
connected case; the stator flux is no longer determined by the grid
voltage and is thus set by regulating the rotor excitation current. A
decoupled orthogonal control using field-oriented techniques can
be used leading to direct control of the stator flux by one of the
rotor current components. The machine equations written in a
synchronously rotating d-q reference frame are:
[3][4][5]:
sqs
sd
sdssd
d
iRv
φω
−+= (1)
sds
sq
sqssq dt
d
iRv
φω
++= (2)
rqr
rd
rdrrd
d
iRv
φω
−+= (3)
rdr
rq
rqrrq dt
d
iRv
φω
++= (4)
srdsdssd MiiL
(5)
0
rqsqssq MiiL
(6)
sdrdrrd MiiL
(7)
sqrqrrq MiiL
(8)