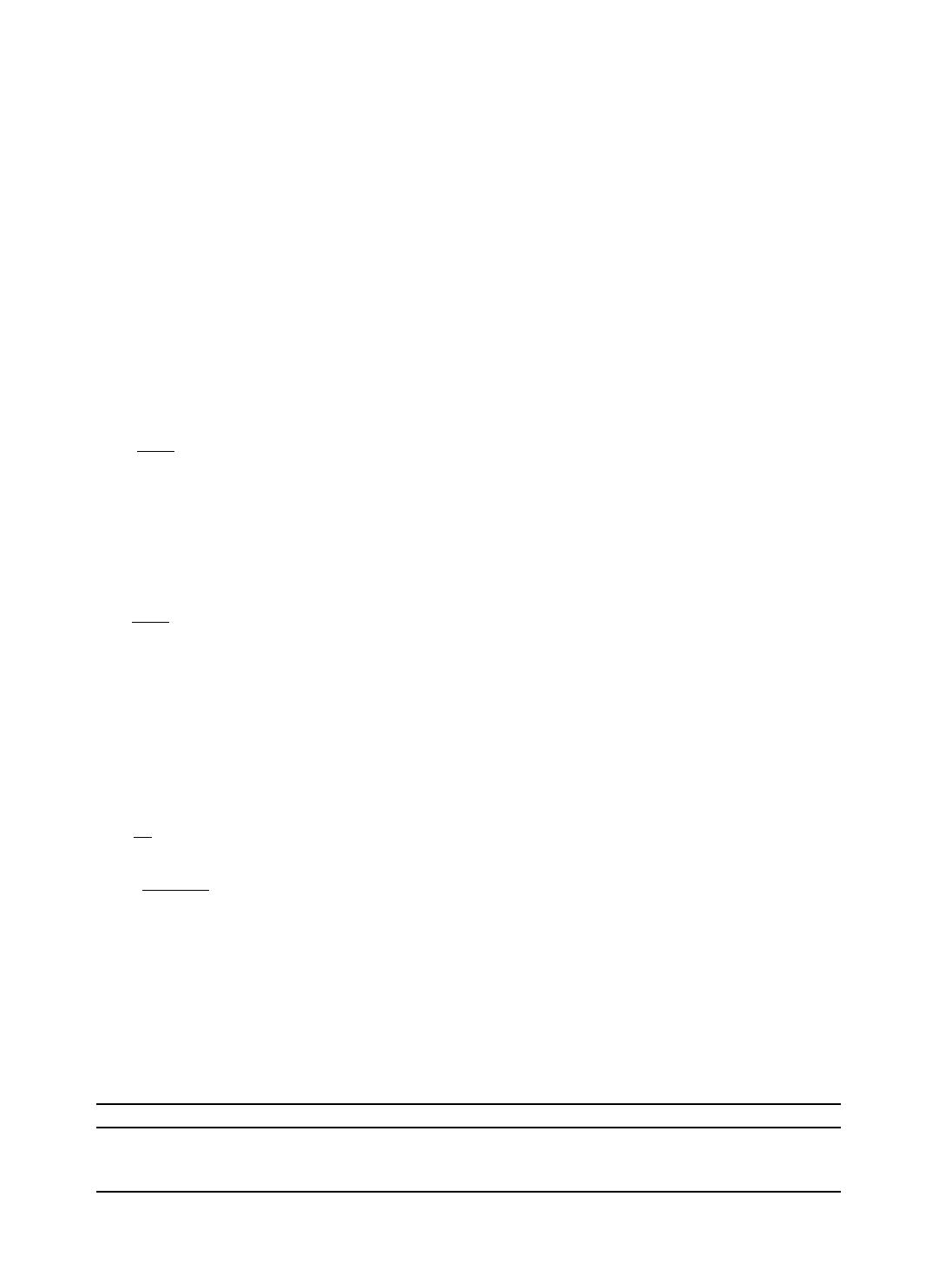
3.2. Xeray diffraction studies
The XRD patterns of spray deposited CZTS thin films deposited at different substrate temperatures (300, 325, 350 and
375
C) are shown in Fig. 2 and prepared CZTS samples are polycrystalline nature with kesterite phase with substrate
temperature [28]. The diffraction peaks at angles 2
q
¼28.49, 47.40 and 56.25
correspond to diffraction planes (112), (220)
and (312), respectively [29] for all samples. Besides, as-prepared thin films might have a complete synthesization without
post-annealing. The intensity and full width at half maximum (FWHM) of (112) peak showed strong and narrow behavior
with good crystallinity.
Fig. 3 gives the relative intensity and full width at half maximum (FWHM) of the (112) peak with respect to substrate
temperature for CZTS films. The relative intensity of the (112) peak continuously increased and FWHM is decreased with
substrate temperature. However, at high substrate temperature (>350
C), the (112) peak intensity dramatically decreased,
shows the weak in crystallinity. Additionally, the lattice parameters of CZTS thin film prepared from 300
Cto375
Care
similar as the other reports [17,21,22]. The lattice parameters have been found to be a ¼5.42 Å and c ¼10.84 Å, which give a
value of c/2a ¼0.99. As the value of c/2a is close to 1 showed the unit cell is tetragonal and enlarged to different substrate
temperature. With the increase of temperature from 300
Cto375
C, the crystallite size increased from 5 to 14 nm, and
decreased the values 7 nm at 375
C substrate temperature. Table 2 shows the microstructural properties of the CZTS thin
films deposited to various substrate temperature. The (hkl) and d-values of CZTS thin films are agree well with the values
found in the JCPDS card [29]. The crystallite size of the films, calculated using Scherrer’s formula [30].
D¼0:9
l
b
cos
q
(1)
where Dis the crystallite size,
b
is the broadening of the diffraction line measured at half of its maximum intensity (rad)
(FWHM) and
l
the X-ray wavelength (1.5418 Å). The prepared CZTS films are polycrystalline in nature. The stresses are one of
the most important critical factors affecting on the structural properties which changes geometric mismatch at boundaries
between crystalline lattices of thin films and substrate [31]. The microstrains (
3
) are developed in the thin films, and
calculated from the following relation [32].
3
¼
b
cos
q
4(2)
The variation of micro structural parameters like microstrain and stacking fault probability to all CZTS samples are shown
in Fig. 4. The value of the interplanar spacing has been increased and internal microstrain relaxation in CZTS thin films is
decreased. The microstrain relaxation is consistent with the expansion of the interplanar spacing of (112) plane and internal
microstrain induces the formation of defect center [33,34]. However, this internal microstrain could be relaxed at the higher
substrate temperature and deposited at low temperature CZTS thin films may be present smaller amounts of defects. The
dislocation density (
d
) and stacking fault probability (
a
) of the CZTS thin films are estimated by the following equations
[35,36].
d
¼1
D2(3)
a
¼2
p
2
45 ffiffiffi
3
ptan
q
D
ð2
q
Þ(4)
Fig. 5 shows the variation of crystallite size (D) and dislocation density (
d
) of CZTS thin films. The dislocation density
decreased from value 3.8 10
16
lines/m
2
to value 0.56 10
16
lines/m
2
and again reached to the value 1.7 10
16
lines/m
2
. The
minimum values are obtained to the CZTS thin film prepared at 350
C substrate temperature. The value of dislocation density
gives the amount of defects in the CZTS thin film, the maximum value of (D) obtained for the CZTS thin film prepared at 350
C
substrate temperature confirms the good crystallinity.
Table 1
The atomic percent of CZTS thin films deposited for different substrate temperature.
Substrate temperature (
C) Cu/(Zn þSn) Zn/Sn Metal/S
300 0.75 1.45 1.40
325 0.76 1.34 1.36
350 0.75 1 1.31
375 0.88 1.19 1.42
S.A. Khalate et al. / Superlattices and Microstructures 103 (2017) 335e342 337