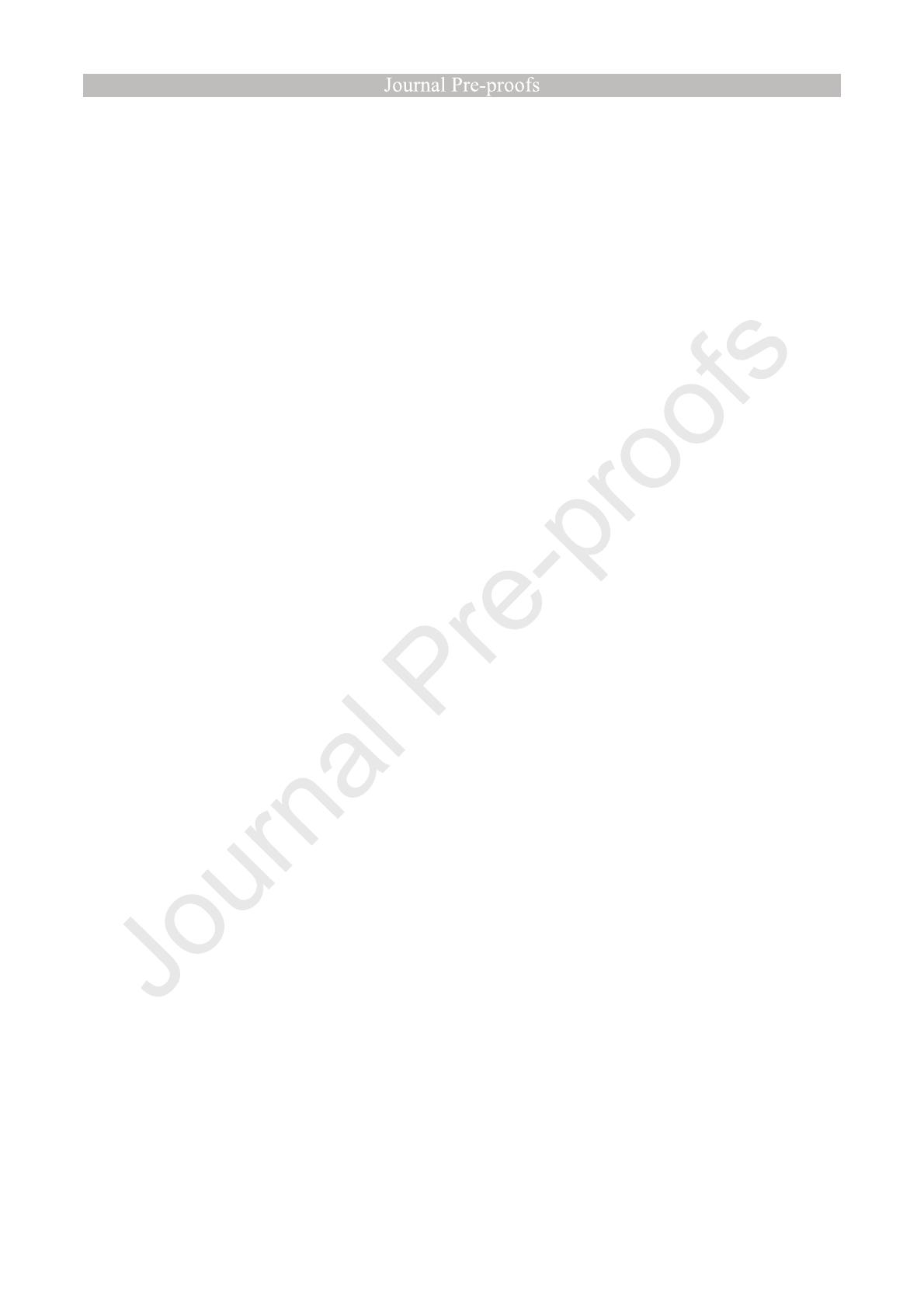
1. Introduction
In last decades, the emergence of flexible electronics for variety of applications, such as
flexible displays, flexible circuits, flexible sensors and flexible solar cells, has led to increased
need for large-scale production processes (Dubal et al., 2018; Lee and Yoo, 2012; Yang and
Gao, 2019). Other than the electronics field, flexible substrates are used in a wide variety of
applications such as membranes, food packaging, artificial skins, microfluidics, etc (Lei and
Wu, 2019; Yang et al., 2019). In general, roll-to-roll systems are preferred to the conventional
batch systems to coat flexible substrates in a continuous manner for the mass production of
many important products (Gao et al., 2016; Guerrero et al., 2011; Maydannik et al., 2011).
When compared with the batch systems, roll-to-roll systems offer significant cost reduction
considering the high yield and large utilized surface area (Kovacik et al., 2015).
The interest in functional polymeric coatings has recently experienced rapid growth
within both the research and industrial community because of their flexible properties (Chen
et al., 2013; Gürsoy et al., 2017b). Functional coatings add desired properties on the surface
of substrates without changing the bulk. Polymer coating processes can be divided into two
main categories, namely wet and dry techniques. In the former ones, the coating material and
a suitable binder are dissolved in a solution that is directly coated on the substrate. The
evaporation of solvent from the wet film results in a dry and stringent coating on substrate
surface. Drop casting, spin coating, dip coating, sol-gel, and spray coating are among the most
utilized coatings for various applications (Danglad-Flores et al., 2018; Schlaich et al., 2018;
Wedershoven et al., 2018). In dry techniques, as its name implies, the usage of solvents is
ruled out, and coating material is directly deposited on the substrate surface through
condensation of vapor-phase precursors (Gürsoy et al., 2019). Physical vapor deposition
(PVD) and chemical vapor deposition (CVD) techniques are widely used dry techniques for
coating thin, conformal, and uniform films on many different types of substrates. Such vapor