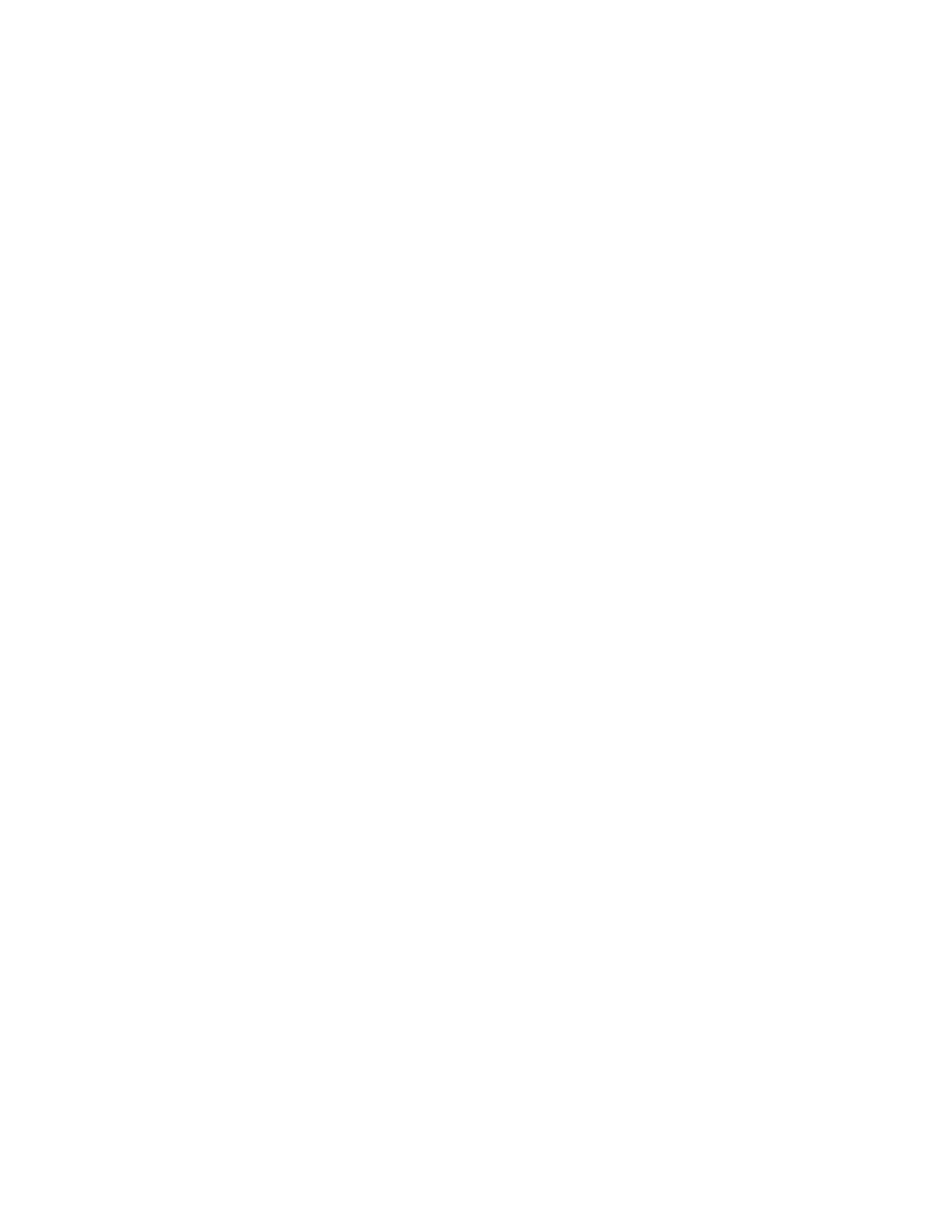
ronmental Monitoring Systems Laboratory, U.S. Environmental
Protection Agency, Cincinnati, Ohio.
2. WETZEL, R.G. & G.E. LIKENS. 1991. Limnological Analyses, 2nd ed.
Springer-Verlag, New York, N.Y.
3. PLANTE, C. & J.A. DOWNING. 1989. Production of freshwater inver-
tebrate populations in lakes. Can. J. Fish. Aquat. Sci. 46:1489.
4. PLANTE, C. & J.A. DOWNING. 1990. Empirical evidence for differ-
ences among methods for calculating secondary production. J. N.
Amer. Benthol. Soc. 9(1):9.
5. GIBSON, G.R., ed. 1996. Biological criteria. In Technical Guidance
for Streams and Small Rivers, EPA-822-B-96-001. Off. Science &
Technology, U.S. Environmental Protection Agency, Washington,
D.C.
6. PLAFKIN, J.L., M.T. BARBOUR, K.D. PORTER, S.K. GROSS & R.M.
HUGHES. 1989. Rapid bioassessment protocols for use in streams and
rivers. In Benthic Macroinvertebrates and Fish, EPA-440/4-89-001.
Off. Water, U.S. Environmental Protection Agency, Washington,
D.C.
7. BARBOUR, M.T., J. GERRITSEN, B.D. SNYDER & J.B. STRIBLING. 1999.
Rapid Bioassessment Protocols for Use in Streams and Wadeable
Rivers: Periphyton, Benthic Macroinvertebrates, and Fish, EPA
841-B-99-002. Off. Water, U.S. Environmental Protection Agency,
Washington, D.C.
8. LAZORCHAK, J.M., D.J. KLEMM & D.V. PECK. 1998. Environmental
Monitoring and Assessment Program-Surface Waters: Field Oper-
ations and Methods for Measuring the Ecological Conditions of
Wadeable Streams, EPA 620-R-94-004F. National Exposure Re-
search Lab., U.S. Environmental Protection Agency, Cincinnati,
Ohio, and National Health & Environmental Effects Research Lab.,
U.S. Environmental Protection Agency, Corvallis, Ore.
9. BARBOUR, M.T. 1997. Perspectives from North America on the
programmatic and scientific elements of an effective bioassessment.
Human Ecol. Risk Assess. 3:929.
4. Bibliography
HYNES, H.B.N. 1963. The Biology of Polluted Waters. Liverpool Univ.
Press, England.
MACAN, T.T. 1963. Freshwater Ecology. John Wiley & Sons, New York,
N.Y.
RUTTNER, F. 1966. Fundamentals of Limnology. Univ. Toronto Press,
Toronto, Ont.
MACKENTHUN, K.M. & W.M. INGRAM. 1967. Biological Associated Prob-
lems in Freshwater Environments. Federal Water Pollution Control
Admin., Washington, D.C.
HYNES, H.B.N. 1970. The Ecology of Running Waters. Univ. Toronto
Press, Toronto, Ont.
ODUM, E.P. 1971. Fundamentals of Ecology, 3rd ed. Saunders Publish-
ing Co., Philadelphia, Pa.
COULL, B.C., ed. 1977. Ecology of Marine Benthos. University of South
Carolina Press, Columbia.
PEARSON,T.H.&R.ROSENBERG. 1978. Macrobenthic succession in
relation to organic enrichment and pollution of the marine environ-
ment. Oceanogr. Mar. Biol. Annu. Rev. 16:229.
BROWER, J.R. & J.H. ZAR. 1984. Field and Laboratory Methods for
General Ecology. Wm. C. Brown Publ., Dubuque, Iowa.
RESH, V.H. & D.M. ROSENBERG. 1984. The Ecology of Aquatic Insects.
Praeger Publ., New York, N.Y.
WARD, J.V. 1984. Ecological perspectives in the management of aquatic
insects. In V.H. Resh & D.M. Rosenberg, eds. The Ecology of
Aquatic Insects, p. 558. Praeger Scientific, New York, N.Y.
WIEDERHOLM, T. 1984. Responses of aquatic insects to environmental
pollution. In V.H. Resh & D.M. Rosenberg, eds. The Ecology of
Aquatic Insects, p. 508. Praeger Scientific, New York, N.Y.
CUMMINS, K.W. & M.A. WILZBACH. 1985. Field Procedures for Analysis
of Functional Feeding Groups of Stream Macroinvertebrates, Con-
tribution 1611. Appalachian Environ. Lab., Univ. Maryland, Frost-
burg.
LEONARD, P.M. & D.J. ORTH. 1986. Application and testing of an index
of biotic integrity in small, coolwater streams. Trans. Amer. Fish.
Soc. 115:401.
WARWICK, R.M. 1986. A new method for determining pollution effects
on marine macrobenthic communities. Mar. Biol. 92:557.
HILSENHOFF, W.L. 1987. An improved biotic index of organic stream
pollution. Great Lakes Entomol. 20:31.
ROHM, C.M., J.W. GIESE & C.C. BENNETT. 1987. Evaluation of aquatic
ecoregion classification of streams in Arkansas. Freshwater Ecol.
4:127.
SHACKELFORD, B. 1988. Rapid Bioassessments of Lotic Macroinverte-
brate Communities: Biocriteria Development. Arkansas Dept. Pol-
lution Control & Ecology, Little Rock.
STEEDMAN, R.J. 1988. Modification and assessment of an index of biotic
integrity to quantify stream quality in southern Ontario. Can. J.
Fish. Aquat. Sci. 45:492.
U.S. ENVIRONMENTAL PROTECTION AGENCY. 1988. Proc. 1st National
Workshop on Biological Criteria, Lincolnwood, Ill., Dec. 2– 4,
1987, Rep. No. 905/9-89/003. U.S. Environmental Protection
Agency, Chicago, Ill.
WHITTIER, T.R., R.M. HUGHES & D.P. LARSEN. 1988. Correspondence
between ecoregions and spatial patterns in stream ecosystems in
Oregon. Can. J. Fish. Aquat. Sci. 45:1264.
OHIO ENVIRONMENTAL PROTECTION AGENCY. 1989. Biological Criteria for
the Protection of Aquatic Life: Volume III. Standardized Biological
Field Sampling and Laboratory Methods for Assessing Fish and
Macroinvertebrate Communities. Ohio Environmental Protection
Agency, Div. Water Quality Monitoring & Assessment, Surface
Water Section, Columbus.
REYNOLDSON, T.B., D.W. SCHLOESSER & B.A. MANNY. 1989. Develop-
ment of a benthic invertebrate objective for mesotrophic Great
Lakes waters. J. Great Lakes Res. 15:669.
BURD, B.J., A. NEMEC & R.O. BRINKHURST. 1990. The development and
application of analytical methods in benthic marine infaunal stud-
ies. Advan. Mar. Biol. 26:169.
NATIONAL RESEARCH COUNCIL. 1990. Managing Troubled Waters: The
Role of Marine Environmental Monitoring. National Academy
Press, Washington, D.C.
WESTON, D. 1990. Quantitative examination of macrobenthic changes
along an organic enrichment gradient. Mar. Ecol. Prog. Ser. 61:
233.
YOUNT, J.D. & G.J. NIEMI. 1990. Recovery of lotic communities and
ecosystems from disturbance—a narrative review of case studies.
Environ. Mgmt. 14:547.
KARR, J.R. & B.L. KERANS. 1992. Components of biological integrity:
their definition and use in development of an invertebrate IBI. In
T.P. Simon & W.S. David, eds. Environmental Indicators: Mea-
surement and Assessment Endpoints, Chapter 1, EPA 905-R-92-
003. U.S. Environmental Protection Agency, Chicago, Ill.
BLAKE,J.A.&A.LISSNER. 1993. Taxonomic atlas of the benthic fauna
of the Santa Maria Basin and Western Santa Barbara Channel, Vol.
1. Santa Barbara Mus. Natural History, Santa Barbara, Calif.
CAIRNS, J., JR. 1993. A proposed framework for developing indicators of
ecosystem health. Hydrobiologia 263:1.
ROSENBERG, D.M. & V.H. RESH, eds. 1993. Freshwater Biomonitoring
and Benthic Macroinvertebrates. Chapman-Hall, New York, N.Y.
GURTZ, M.E. 1994. Design of biological components of the National
Water-Quality Assessment (NAWQA) Program. In S.L. Loeb & A.
Spacie, eds. Biological Monitoring of Aquatic Systems. Lewis
Publishers, Ann Arbor, Mich.
DAVIS, W.S. & T.P. SIMON, eds. 1995. Biological Assessment and
Criteria: Tools for Water Resource Planning and Decision Making.
Lewis Publishers, Boca Raton, Fla.
BENTHIC MACROINVERTEBRATES (10500)/Introduction
2
BENTHIC MACROINVERTEBRATES (10500)/Introduction