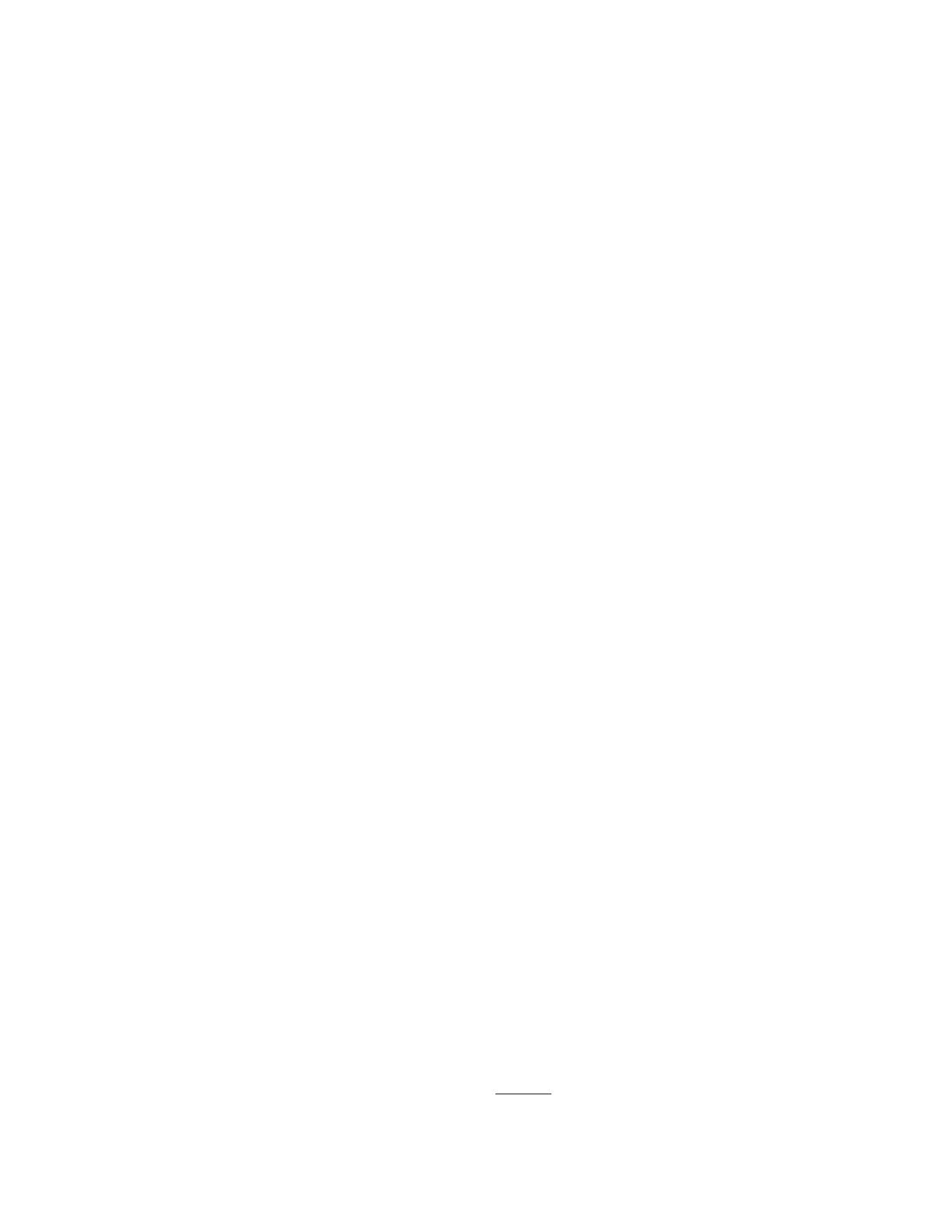
addition of known quantities of standard. Repeat procedure for
sample. If the slope of the stripping current versus added metal
is significantly different in the sample relative to the metal-free
solution, digest sample further. The choice of stripping wave-
form also is important. While both square-wave and differential-
pulse stripping attempt to minimize the contribution of adsorp-
tion currents to the total measured stripping current, square-wave
stripping does this more effectively. Thus, use square-wave
stripping instead of differential-pulse stripping when adsorption
occurs.
2.
Apparatus
a. Electrochemical analyzer: The basic electrochemical ana-
lyzer for ASV applications contains a three-electrode poten-
tiostat, which very precisely controls potential applied to the
working electrode relative to the reference electrode, and a
sensitive current measuring device. It is capable of delivering
potential pulses of various amplitudes and frequencies, and pro-
vides several scan rates and current ranges. More advanced ASV
instruments offer automated timing, gas purge and stirring, and
data processing routines including curve smoothing, baseline
correction, and background subtraction.
Two variations of stripping waveforms are commonly used:
differential pulse (DPASV) and square wave (SWASV) wave-
forms. The differential-pulse waveform consists of a series of
pulses superimposed on a linear voltage ramp, while the square-
wave waveform consists of a series of pulses superimposed on a
staircase potential waveform. Square-wave stripping is signifi-
cantly faster than differential-pulse stripping and is typically ten
times more sensitive. Most commercially available ASV instru-
ments perform both differential-pulse and square-wave stripping.
b. Electrodes and cell: Provide working, reference, and aux-
iliary electrodes. Working electrodes are either hanging mercury
drop or thin mercury film electrodes. Hanging mercury drop
electrodes must be capable of dispensing mercury in very pre-
cisely controlled drop sizes. Three types of electrodes meet this
requirement: static mercury drop electrodes, controlled growth
mercury drop electrodes, or Kemula-type electrodes. In any case,
use a drop knocker to remove an old drop before dispensing a
fresh mercury drop.
When the lowest detection levels are required, a thin mercury
film electrode is preferred. This electrode consists of a rotating
glassy carbon disk plated with mercury in situ during precon-
centration of the analyte. A high-precision, constant-speed rota-
tor controls the rotation rate of the electrode and provides repro-
ducible mass transport.
Reference electrodes may be either saturated calomel or silver/silver
chloride electrodes. Use a platinum wire for the auxiliary electrode.
Use cells constructed of glass, or preferably fused silica or
TFE, because they are more resistant to solution adsorption or
leaching. Cover cell with a lid that provides reproducible place-
ment of the electrodes and gas purging tubes. Provide an addi-
tional hole in the cell lid for addition of standards. Most com-
mercially available mercury drop electrodes include electrolytic
cells, reference and auxiliary electrodes, and gas purging tubes.
Use a constant-speed stirring mechanism to provide reproduc-
ible mass transport in samples and standards.
Locate the cell in an area where temperature is relatively constant.
Alternatively, use a constant-temperature water bath and cell jacket.
c. Oxygen-removal apparatus: Oxygen interferes in electro-
chemical analyses; remove it from solution before preconcentra-
tion by purging with nitrogen or argon. Provide two gas inlet
tubes through the cell lid: one extends into the solution and the
second purges the space above the solution. A gas outlet hole in
the lid provides for removal of oxygen and excess purging gas.
d. Recording device: If the electrochemical analyzer is not
equipped with a digital data acquisition system, use an XY
plotter to record stripping voltammograms.
e. Timer: If preconcentration and equilibration periods are not
controlled by the instrument, use an accurate timing device.
f. Polishing wheel: To obtain the high polish required for a
glassy carbon disk electrode, use a motorized polishing wheel.
3.
Reagents
Wherever possible, use high-purity reagents that conform to
ACS specifications.
CAUTION:Follow proper practices for disposal of any solu-
tions containing mercury.
a. Metal-free water: Use deionized water to prepare buffers,
electrolytes, standards, etc. Use water with at least 18
megohm-cm resistivity (see Section 1080).
b. Nitric acid, HNO
3
, conc, high-purity.*
c. Nitric acid, HNO
3
,6N, 1.6N(10%), and 0.01N.
d. Purging gas (nitrogen or argon), high-purity. Remove
traces of oxygen in nitrogen or argon gas before purging the
solution. Pass gas through sequential scrubbing columns con-
taining vanadous chloride in the first, deionized water in the
second, and buffer (or electrolyte) solution in the third column.
e. Metal standards: Prepare stock solutions containing 1 mg
metal/mL in polyethylene bottles. Purchase these solutions com-
mercially or prepare as in Section 3111. Daily prepare dilutions
of stock standards in a matrix similar to that of the samples to
cover the concentration range desired.
f. Electrolyte/buffer: Use one of the following:
1) Acetate buffer, pH 4.5: Dissolve 16.4 g anhydrous sodium
acetate, NaC
2
H
3
O
2
, in 800 mL water. Adjust to pH 4.5 with
high-purity glacial acetic acid.* Dilute to 1 L with water.
2) Citrate buffer, pH 3: Dissolve 42.5 g citric acid (monohy-
drate) in 700 mL water. Adjust to pH 3 with high-purity
NH
4
OH.* Dilute to 1 L with water.
3) Phosphate buffer, pH 6.8: Dissolve 24 g NaH
2
PO
4
in 500
mL water. Adjust to pH 6.8 with 1NNaOH. Dilute to 1 L with
water.
g. Mercury: Use commercially available triply distilled me-
tallic mercury for hanging mercury drop electrodes. CAUTION:
Mercury vapors are highly toxic. Use only in well-ventilated
area.
h. Mercuric nitrate solution, Hg(NO
3
)
2
: For thin mercury film
electrodes, dissolve 0.325 g Hg(NO
3
)
2
in 100 mL 0.01NHNO
3
.
i. Reference electrode filling solution: Available from elec-
trode manufacturer.
j. Amalgamated zinc: Dissolve 2 g Hg(NO
3
)
2
in 25 mL conc
HNO
3
; dilute to 250 mL with water. In a separate beaker, clean
approximately 50 g mossy zinc by gently oxidizing the surface
with 10% HNO
3
and rinse with water. Add Hg(NO
3
)
2
solution to
* Ultrex, Suprapur, Aristar, or equivalent.
ANODIC STRIPPING VOLTAMMETRY (3130)/Determination of Lead, Cadmium, and Zinc
2
ANODIC STRIPPING VOLTAMMETRY (3130)/Determination of Lead, Cadmium, and Zinc