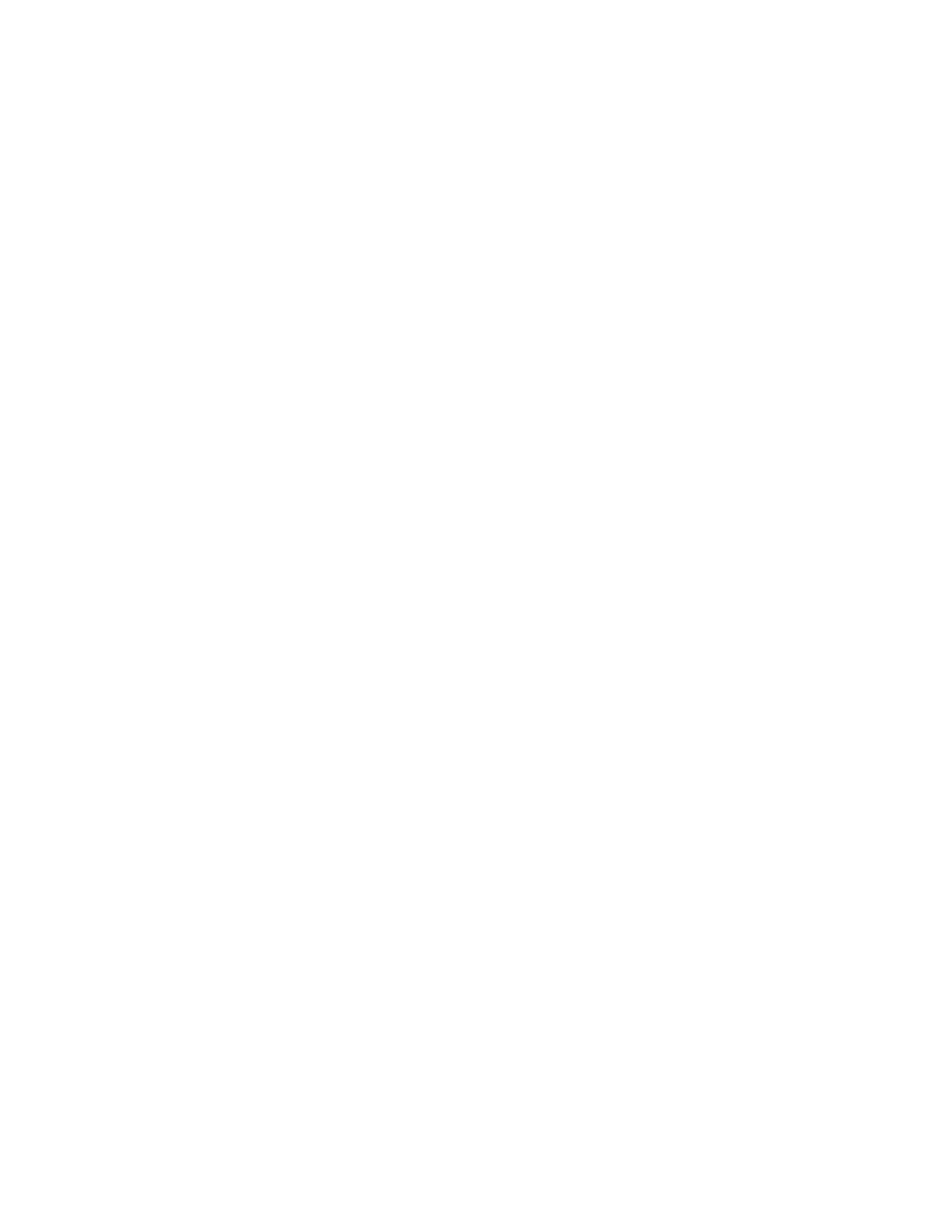
manufacturer’s recommendations.† Report column and packing
specifications and conditions of analyses with results.
A two-column system usually is required. A molecular sieve
column separates H
2
,O
2
,N
2
, CO, and CH
4
isothermally, but
because CO
2
is adsorbed by the molecular sieve, a second
column is needed to complete the analysis. A commonly used
two-column system utilizes a Chromosorb 102 column and a
Molecular Sieve 5A or 13X column to separate H
2
,O
2
,N
2
,CH
4
,
and CO
2
isothermally.
1,2
A single-column procedure can be used; however, it requires
temperature programming. Columns packed with special porous
spherical or granular packing materials effect separation with
sharp, well-resolved peaks.
3
With temperature programming,
columns packed with Chromosorb 102,
2
Carbosphere,
4
and Car-
bosieve
3
separate the gases listed.† Commercial equipment spe-
cifically designed for such operations is available.
2,4–6
d. Integrator/recorder: Use a 10-mV full-span strip chart
recorder with the gas chromatograph. When minor components
such as H
2
and H
2
S are to be detected, a 1-mV full-span recorder
is preferable.
Integrators that can easily detect very minute quantities of gases
are available. Computerized data-processing systems, to record and
manipulate the chromatographic signal, chromatographic base line,
etc., also are available.
3.
Reagents
a. Carrier gases: Preferably use helium for separating digester
gases. It is impossible to detect less than 1% hydrogen when helium
is used as the carrier gas.
1
Obtain linear TCD responses for molar
concentrations of hydrogen between 0 and 60% by using an 8.5%
hydrogen–91.5% helium carrier gas mixture.
7
To detect trace quan-
tities of hydrogen use argon or nitrogen as carrier gas.
1
b. Calibration gases: Use samples of CH
4
,CO
2
, and N
2
of
known purity, or gas mixtures of certified composition, for calibra-
tion. Also use samples of O
2
,H
2
, and H
2
S of known purity if these
gases are to be measured. Preferably use custom-made gas mixtures
to closely approximate digester gas composition.
c. Displacement liquids: See 2720B.3d.
4.
Procedure
a. Preparation of gas chromatograph: Open main valve of car-
rier-gas cylinder and adjust carrier-gas flow rate to recommended
values. To obtain accurate flow measurements, connect a soap-film
flow meter to the TCD vent. Turn power on. Turn on oven heaters,
if used, and detector current and adjust to desired values.
Set injection port and column temperatures as specified for the
column being used. Set TCD current. Turn on recorder or data
processor. Check that the injector/detector temperature has risen
to the appropriate level and confirm that column temperature is
stabilized. Set range and attenuation at appropriate positions.
The instrument is ready for use when the recorder yields a
stable base line. Silica gel and molecular sieve columns gradu-
ally lose activity because of adsorbed moisture or materials
permanently adsorbed at room temperature. If insufficient sepa-
rations occur, reactivate by heating or repacking.
b. Calibration: For accurate results, prepare a calibration curve
for each gas to be measured because different gas components do
not give equivalent detector responses on either a weight or a molar
basis. Calibrate with synthetic mixtures or with pure gases.
1) Synthetic mixtures—Use purchased gas mixtures of certi-
fied composition or prepare in the laboratory. Inject a standard
volume of each mixture into the gas chromatograph and note
response for each gas. Compute detector response, either as area
under a peak or as height of peak, after correcting for attenua-
tion. Read peak heights accurately and correlate with concentra-
tion of component in sample. Reproduce operating parameters
exactly from one analysis to the next. If sufficient reproducibility
cannot be obtained by this procedure, use peak areas for cali-
bration. Preferably use peak areas when peaks are not symmet-
rical. Prepare calibration curve by plotting either peak area or
peak height against volume or mole percent for each component.
Modern integrators and data-processing systems are able to
generate calibration tables for certified gas mixtures.
2) Pure gases—Introduce pure gases into chromatograph in-
dividually with a syringe. Inject sample volumes of 0.25, 0.5, 1.0
mL, etc., and plot detector response, corrected for attenuation,
against gas volume.
When the analysis system yields a linear detector response
with increasing gas component concentration from zero to the
range of interest, run standard mixtures along with samples. If
the same sample size is used, calculate gas concentration by
direct proportions.
c. Sample analysis: If samples are to be injected with a
syringe, equip sample collection container with a port closed by
a rubber or silicone septum. To take a sample for analysis, expel
air from barrel of syringe by depressing plunger and force needle
through the septum. Withdraw plunger to take gas volume de-
sired, pull needle from collection container, and inject sample
rapidly into chromatograph.
When samples are to be injected through a gas-sampling
valve, connect sample collection container to inlet tube. Let gas
flow from collection tube through the valve to purge dead air
space and fill sample tube. About 15 mL normally are sufficient
to clear the lines and to provide a sample of 1 to 2 mL. Transfer
sample from loop into carrier gas stream by following manufac-
turer’s instructions. Bring samples to atmospheric pressure be-
fore injection.
When calibration curves have been prepared with a synthetic
gas mixture of certified composition, use the same sample vol-
ume as that used during calibration. When calibration curves are
prepared by the procedure using varying volumes of pure gases,
inject any convenient gas sample volume up to about 2 mL.
Inject sample and standard gases in sequence to permit calcu-
lation of unknown gas concentration in volume (or mole) percent
by direct comparison of sample and standard gas peak heights or
areas. For more accurate analysis, make duplicate or triplicate
injections of sample and standard gases.
5.
Calculation
a. When calibration curves have been prepared with synthetic
mixtures and the volume of the sample analyzed is the same as
that used in calibration, read volume percent of each component
directly from calibration curve after detector response for that
component is computed.
ANAEROBIC SLUDE DIGESTER GAS (2720) (2720)/Gas Chromatographic Method
4
ANAEROBIC SLUDE DIGESTER GAS (2720) (2720)/Gas Chromatographic Method